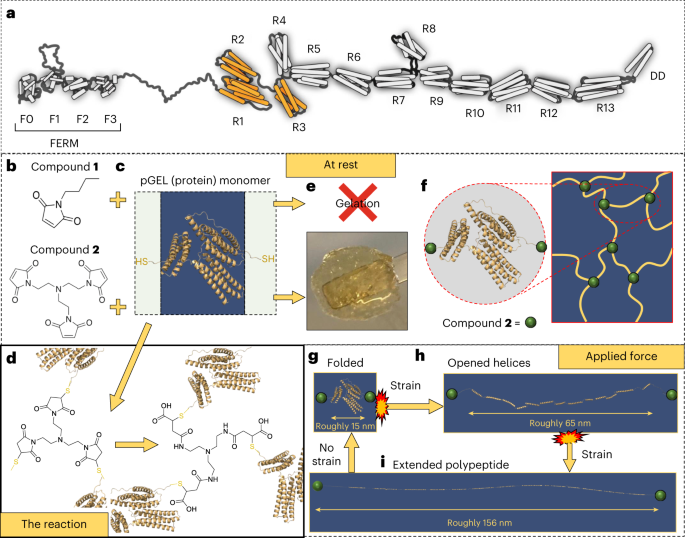
Qiao, P. Z., Yang, M. J. & Bobaru, F. Impact mechanics and high-energy absorbing materials: review. J. Aerosp. Eng. 21, 235–248 (2008).
Dyatkin, B. US hypersonics initiatives require accelerated efforts of the materials research community. MRS Bull. 46, 201–203 (2021).
Viana, J. C. Polymeric materials for impact and energy dissipation. Plast. Rubber Compos. 35, 260–267 (2006).
Park, J. L., Chi, Y. S., Hahn, M. H. & Kang, T. J. Kinetic dissipation in ballistic tests of soft body armors. Exp. Mech. 52, 1239–1250 (2012).
Fejdys, M., Kosla, K., Kucharska-Jastrzabek, A. & Landwijt, M. Influence of ceramic properties on the ballistic performance of the hybrid ceramic-multi-layered UHMWPE composite armour. J. Aust. Ceram. Soc. 57, 149–161 (2021).
Reis, R. H. M. et al. Ballistic performance of guaruman fiber composites in multilayered armor system and as single target. Polymers 13, 1203 (2021).
Wen, Y. K., Xu, C., Wang, S. & Batra, R. C. Analysis of behind the armor ballistic trauma. J. Mech. Behav. Biomed. Mater. 45, 11–21 (2015).
Kearsley, A. T. How laboratory hypervelocity impact experiments have helped us to understand comet dust samples: a brief review. Procedia Eng. 204, 43–50 (2017).
Woignier, T., Duffours, L., Colombel, P. & Durin, C. Aerogels materials as space debris collectors. Adv. Mater. Sci. Eng. 2013, 484153 (2013).
Jones, S. M., Anderson, M. S., Dominguez, G. & Tsapin, A. Thermal calibrations of hypervelocity capture in aerogel using magnetic iron oxide particles. Icarus 226, 1–9 (2013).
Alwin, S. & Shajan, X. S. Aerogels: promising nanostructured materials for energy conversion and storage applications. Mater. Renew. Sustain. Energy 9, 7 (2020).
Bheekhun, N., Abu Talib, A. & Hassan, M. R. Aerogels in aerospace: an overview. Adv. Mater. Sci. Eng. 2013, 406065 (2013).
Kan, A. & Joshi, N. S. Towards the directed evolution of protein materials. MRS Commun. 9, 441–455 (2019).
Wu, J. H. et al. Rationally designed synthetic protein hydrogels with predictable mechanical properties. Nat. Commun. 9, 620 (2018).
Fang, J. et al. Forced protein unfolding leads to highly elastic and tough protein hydrogels. Nat. Commun. 4, 2974 (2013).
Zhu, F. B. et al. 3D-printed ultratough hydrogel structures with titin-like domains. ACS Appl. Mater. Interfaces 9, 11363–11367 (2017).
Bate, N. et al. Talin contains a C-terminal calpain2 cleavage site important in focal adhesion dynamics. PLoS ONE 7, e34461 (2012).
Yao, M. X. et al. The mechanical response of talin. Nat. Commun. 7, 11966 (2016).
Funtan, S., Michael, P. & Binder, W. H. Synthesis and mechanochemical activity of peptide-based Cu(I) bis(N-heterocyclic carbene) complexes. Biomimetics 4, 24 (2019).
Goult, B. T. et al. RIAM and vinculin binding to talin are mutually exclusive and regulate adhesion assembly and turnover. J. Biol. Chem. 288, 8238–8249 (2013).
Schon, A., Clarkson, B. R., Jaime, M. & Freire, E. Temperature stability of proteins: analysis of irreversible denaturation using isothermal calorimetry. Proteins Struct. Funct. Bioinform. 85, 2009–2016 (2017).
Jackson, M. & Mantsch, H. H. The use and misuse of FTIR spectroscopy in the determination of protein-structure. Crit. Rev. Biochem. Mol. Biol. 30, 95–120 (1995).
Öhrlund, Å. Evaluation of rheometry amplitude sweep cross-over point as an index of flexibility for HA fillers. J. Cosmet. Dermatol. Sci. Appl. 8, 47–54 (2018).
Kulkarni, V. S. & Shaw, C. in Essential Chemistry for Formulators of Semisolid and Liquid Dosages (eds Kulkarni, V. S. & Shaw, C.) 145–182 (Academic Press, 2016).
Wen, Q. & Janmey, P. A. Polymer physics of the cytoskeleton. Curr. Opin. Solid State Mater. Sci. 15, 177–182 (2011).
Yao, M. X. et al. Mechanical activation of vinculin binding to talin locks talin in an unfolded conformation. Sci. Rep. 4, 4610 (2014).
Williamsen, J., Pechkis, D., Balakrishnan, A. & Ouelette, S. In Proc. First International Orbital Debris Conference (Lunar and Planetary Institute, 2019).
Couldrick, C. in Advances in Military Textiles and Personal Equipment (ed. Sparks, E.) 196–212 (Woodhead Publishing, 2012).
Veysset, D. et al. High-velocity micro-particle impact on gelatin and synthetic hydrogel. J. Mech. Behav. Biomed. Mater. 86, 71–76 (2018).
Kokol, V., Pottathara, Y. B., Mihelčič, M. & Perše, L. S. Rheological properties of gelatine hydrogels affected by flow- and horizontally-induced cooling rates during 3D cryo-printing. Colloids Surf. A 616, 126356 (2021).
Barnett, S. F. H. & Goult, B. T. The MeshCODE to scale–visualising synaptic binary information. Front. Cell. Neurosci. 16, 1014629 (2022).
Dedden, D. et al. The architecture of Talin1 reveals an autoinhibition mechanism. Cell 179, 120–131 (2019).
Fillingham, I. et al. A vinculin binding domain from the talin rod unfolds to form a complex with the vinculin head. Structure 13, 65–74 (2005).
Burchell, M. J., Cole, M. J., McDonnell, J. A. M. & Zarnecki, J. C. Hypervelocity impact studies using the 2 MV Van de Graaff accelerator and two-stage light gas gun of the University of Kent at Canterbury. Meas. Sci. Technol. 10, 41–50 (1999).
Hibbert, R., Cole, M. J., Price, M. C. & Burchell, M. J. The hypervelocity impact facility at the University of Kent: recent upgrades and specialized capabilities. Procedia Eng. 204, 208–214 (2017).
- SEO Powered Content & PR Distribution. Get Amplified Today.
- PlatoData.Network Vertical Generative Ai. Empower Yourself. Access Here.
- PlatoAiStream. Web3 Intelligence. Knowledge Amplified. Access Here.
- PlatoESG. Automotive / EVs, Carbon, CleanTech, Energy, Environment, Solar, Waste Management. Access Here.
- BlockOffsets. Modernizing Environmental Offset Ownership. Access Here.
- Source: https://www.nature.com/articles/s41565-023-01431-1
- ][p
- 1
- 10
- 11
- 12
- 13
- 14
- 15%
- 16
- 17
- 19
- 1999
- 20
- 2005
- 2006
- 2008
- 2011
- 2012
- 2013
- 2014
- 2015
- 2016
- 2017
- 2018
- 2019
- 2020
- 2021
- 2022
- 22
- 23
- 24
- 25
- 26
- 27
- 28
- 30
- 31
- 32
- 3d
- 7
- 75
- 8
- 9
- a
- academic
- accelerated
- accelerator
- Activation
- activity
- Aerospace
- AL
- an
- analysis
- and
- anderson
- applications
- architecture
- ARE
- article
- AS
- Assembly
- At
- behind
- binding
- body
- bull
- by
- capabilities
- capture
- cell
- chemistry
- click
- collectors
- community
- complex
- contains
- Conversion
- designed
- determination
- domain
- domains
- during
- Dust
- dynamics
- e
- E&T
- ed
- efforts
- energy
- Ether (ETH)
- evaluation
- evolution
- Exclusive
- experiments
- Facility
- First
- Flexibility
- For
- form
- from
- GAS
- Have
- head
- helped
- highly
- How
- http
- HTTPS
- Hybrid
- i
- Impact
- Impacts
- important
- in
- index
- influence
- information
- initiatives
- Institute
- International
- Joshi
- laboratory
- Leads
- light
- LINK
- Liquid
- Locks
- Lunar
- materials
- Materials for energy
- mechanical
- mechanics
- mechanism
- Michael
- Military
- MOL
- MultiLayered
- mutually
- nanotechnology
- Nature
- next-generation
- of
- on
- overview
- performance
- personal
- Physics
- plato
- Plato Data Intelligence
- PlatoData
- Point
- polymer
- Predictable
- press
- price
- promising
- properties
- Protein
- Proteins
- Publishing
- Rates
- recent
- Regulate
- require
- research
- Research Community
- response
- Reveals
- review
- rubber
- s
- SCI
- single
- site
- Soft
- solid
- Space
- Sparks
- specialized
- Spectroscopy
- Stability
- State
- storage
- studies
- supersonic
- surf
- Sweep
- synthetic
- system
- Target
- tests
- textiles
- The
- thermal
- to
- tough
- towards
- turnover
- understand
- unfolding
- university
- upgrades
- us
- use
- using
- W
- with
- X
- zephyrnet