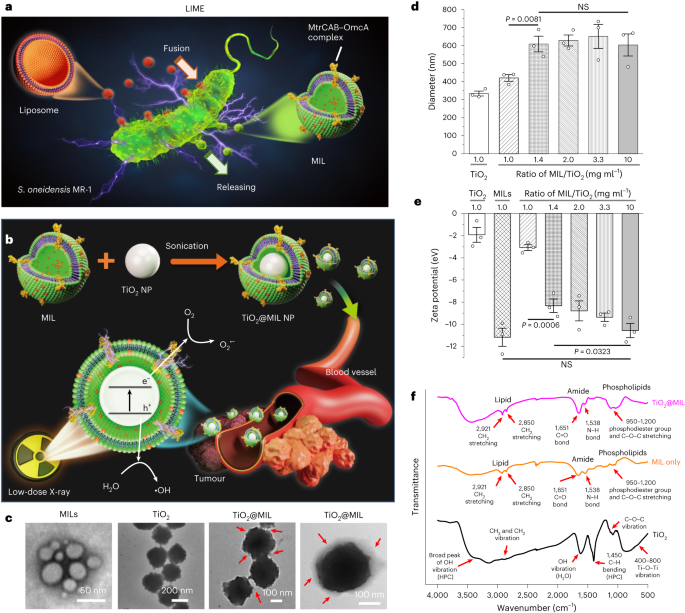
Shi, L. et al. Extracellular electron transfer mechanisms between microorganisms and minerals. Nat. Rev. Microbiol. 14, 651–662 (2016).
Silhavy, T. J., Kahne, D. & Walker, S. The bacterial cell envelope. CSH Perspect. Biol. 2, a000414 (2010).
Castelle, C. et al. A new iron-oxidizing/O2-reducing supercomplex spanning both inner and outer membranes, isolated from the extreme acidophile Acidithiobacillus ferrooxidans. J. Biol. Chem. 283, 25803–25811 (2008).
Beliaev, A. S., Saffarini, D. A., McLaughlin, J. L. & Hunnicutt, D. MtrC, an outer membrane decahaem c cytochrome required for metal reduction in Shewanella putrefaciens MR-1. Mol. Microbiol. 39, 722–730 (2001).
Myers, C. R. & Myers, J. M. MtrB is required for proper incorporation of the cytochromes OmcA and OmcB into the outer membrane of Shewanella putrefaciens MR-1. Appl. Environ. Microb. 68, 5585–5594 (2002).
Bretschger, O. et al. Current production and metal oxide reduction by Shewanella oneidensis MR-1 wild type and mutants. Appl. Environ. Microbiol. 73, 7003–7012 (2007).
Edwards, M. J., White, G. F., Butt, J. N., Richardson, D. J. & Clarke, T. A. The crystal structure of a biological insulated transmembrane molecular wire. Cell 181, 665–673 (2020).
Choi, S. Electrogenic bacteria promise new opportunities for powering, sensing, and synthesizing. Small 18, 2107902 (2022).
Ainsworth, E. V. et al. Photoreduction of Shewanella oneidensis extracellular cytochromes by organic chromophores and dye-sensitized TiO2. ChemBioChem 17, 2324–2333 (2016).
Liu, L. & Choi, S. Enhanced biophotoelectricity generation in cyanobacterial biophotovoltaics with intracellularly biosynthesized gold nanoparticles. J. Power Sources 506, 230251 (2021).
Deng, X., Luo, D. & Okamoto, A. Defined and unknown roles of conductive nanoparticles for the enhancement of microbial current generation: a review. Bioresour. Technol. 350, 126844 (2022).
Chen, Q. W. et al. Self-mineralized photothermal bacteria hybridizing with mitochondria-targeted metal–organic frameworks for augmenting photothermal tumor therapy. Adv. Funct. Mater. 30, 1909806 (2020).
Wang, J. W. et al. A self-driven bioreactor based on bacterium–metal–organic framework biohybrids for boosting chemotherapy via lactate catabolism. ACS Nano 15, 17870–17884 (2021).
Wang, X. N., Niu, M. T., Fan, J. X., Chen, Q. W. & Zhang, X. Z. Photoelectric bacteria enhance the in situ production of tetrodotoxin for antitumor therapy. Nano Lett. 21, 4270–4279 (2021).
Li, W. P., Long, X., Kataoka-Hamai, C. & Okamoto, A. Membrane integrated liposome synthesized by a liposome fusion-induced membrane exchange. Preprint at https://doi.org/10.26434/chemrxiv-2022-9tt9m (2022).
Choi, W., Choi, J. Y. & Song, H. Regulation of electron–hole recombination kinetics on uniform metal–semiconductor nanostructures for photocatalytic hydrogen evolution. APL Mater. 7, 100702 (2019).
Chen, L. et al. Composition tunability of semiconductor radiosensitizers for low-dose X-ray induced photodynamic therapy. J. Nanobiotechnol. 20, 293 (2022).
Moon, J. T., Lee, S. K. & Joo, J. B. Controllable one-pot synthesis of uniform colloidal TiO2 particles in a mixed solvent solution for photocatalysis. Beilstein J. Nanotechnol. 9, 1715–1727 (2018).
Zhu, L. J. et al. Ligand-free rutile and anatase TiO2 nanocrystals as electron extraction layers for high performance inverted polymer solar cells. RSC Adv. 7, 20084–20092 (2017).
Mihaly, J. et al. Characterization of extracellular vesicles by IR spectroscopy: fast and simple classification based on amide and C–H stretching vibrations. Biochim. Biophys. Acta Biomembr. 1859, 459–466 (2017).
Bonechi, C. et al. Physicochemical characterization of hyaluronic acid and chitosan liposome. Coat. Appl. Sci. 11, 12071 (2021).
Reuillard, B. et al. High performance reduction of H2O2 with an electron transport decaheme cytochrome on a porous ITO electrode. J. Am. Chem. Soc. 139, 3324–3327 (2017).
Okamoto, A., Nakamura, R. & Hashimoto, K. In-vivo identification of direct electron transfer from Shewanella oneidensis MR-1 to electrodes via outer-membrane OmcA–MtrCAB protein complexes. Electrochim. Acta 56, 5526–5531 (2011).
Okamoto, A., Tokunou, Y., Kalathil, S. & Hashimoto, K. Proton transport in the outer-membrane flavocytochrome complex limits the rate of extracellular electron transport. Angew. Chem. Int. Ed. 56, 9082–9086 (2017).
Van Acker, H. et al. The role of reactive oxygen species in antibiotic-induced cell death in Burkholderia cepacia complex bacteria. PLoS ONE 11, e0159837 (2016).
Shoji, T. et al. DMPO–OH radical formation from 5,5-dimethyl-1-pyrroline N-oxide (DMPO) in hot water. Anal. Sci. 23, 219–221 (2007).
Fridovich, I. Superoxide radical and superoxide dismutases. Annu. Rev. Biochem. 64, 97–112 (1995).
Oberley, L. W. & Buettner, G. R. Role of superoxide-dismutase in cancer—review. Cancer Res. 39, 1141–1149 (1979).
Marklund, S. L., Westman, N. G., Lundgren, E. & Roos, G. Copper-containing and zinc-containing superoxide-dismutase, manganese-containing superoxide-dismutase, catalase, and glutathione-peroxidase in normal and neoplastic human cell-lines and normal human-tissues. Cancer Res. 42, 1955–1961 (1982).
Huang, P., Feng, L., Oldham, E. A., Keating, M. J. & Plunkett, W. Superoxide dismutase as a target for the selective killing of cancer cells. Nature 407, 390–395 (2000).
Clark, J. M. The 3Rs in research: a contemporary approach to replacement, reduction and refinement. Br. J. Nutr. 120, S1–S7 (2018).
- SEO Powered Content & PR Distribution. Get Amplified Today.
- PlatoData.Network Vertical Generative Ai. Empower Yourself. Access Here.
- PlatoAiStream. Web3 Intelligence. Knowledge Amplified. Access Here.
- PlatoESG. Automotive / EVs, Carbon, CleanTech, Energy, Environment, Solar, Waste Management. Access Here.
- BlockOffsets. Modernizing Environmental Offset Ownership. Access Here.
- Source: https://www.nature.com/articles/s41565-023-01476-2
- :is
- ][p
- 1
- 10
- 11
- 12
- 13
- 14
- 15%
- 16
- 17
- 19
- 20
- 2000
- 2001
- 2008
- 2011
- 2016
- 2017
- 2018
- 2019
- 2020
- 2021
- 2022
- 22
- 23
- 24
- 25
- 26
- 27
- 28
- 30
- 31
- 32
- 65
- 7
- 8
- 9
- a
- AL
- am
- an
- and
- approach
- article
- AS
- At
- b
- Bacteria
- based
- between
- boosting
- both
- by
- Cancer
- Cancer cells
- cell
- Cells
- chemotherapy
- chen
- classification
- click
- complex
- composition
- contemporary
- Crystal
- Current
- Death
- defined
- direct
- e
- E&T
- ed
- enhance
- enhanced
- enhancement
- Ether (ETH)
- evolution
- exchange
- extraction
- extreme
- fan
- FAST
- For
- formation
- Framework
- frameworks
- from
- generation
- Gold
- High
- HOT
- http
- HTTPS
- human
- hydrogen
- i
- Identification
- in
- increased
- integrated
- into
- isolated
- layers
- Lee
- limits
- LINK
- Long
- mechanisms
- metal
- minerals
- mixed
- molecular
- nanotechnology
- Nature
- New
- normal
- of
- on
- opportunities
- organic
- Oxygen
- performance
- plato
- Plato Data Intelligence
- PlatoData
- polymer
- power
- Powering
- Production
- promise
- proper
- Protein
- R
- radical
- Rate
- reduction
- Regulation
- replacement
- required
- research
- review
- Role
- roles
- s
- Scholar
- SCI
- selective
- semiconductor
- Simple
- solar
- Solar cells
- solution
- song
- Spectroscopy
- structure
- T
- Target
- The
- therapy
- to
- transfer
- transport
- tumor
- type
- unknown
- via
- W
- walker
- Water
- white
- Wild
- Wire
- with
- X
- x-ray
- zephyrnet