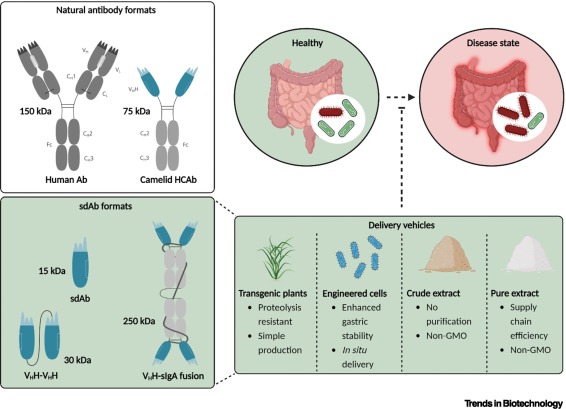
Global Action Plan on Antimicrobial Resistance.
World Health Organization, 2015
Changing priorities in vaccinology: antibiotic resistance moving to the top.
Front. Immunol. 2018; 9: 1068
Maternal antibodies: clinical significance, mechanism of interference with immune responses, and possible vaccination strategies.
Front. Immunol. 2014; 5: 446
Passive immunization.
Plotkins Vacc. 2018; 2018: 85-95
Changes in human milk immunoglobulin profile during prolonged lactation.
Front. Pediatr. 2020; 8: 428
Animal immunization, in vitro display technologies, and machine learning for antibody discovery.
Trends Biotechnol. 2021; 39: 1263-1273
Fully human VH single domains that rival the stability and cleft recognition of camelid antibodies.
J. Biol. Chem. 2015; 290: 11905-11917
Applications of nanobodies.
Annu. Rev. Anim. Biosci. 2021; 9: 401-421
Nanobodies: natural single-domain antibodies.
Annu. Rev. Biochem. 2013; 82: 775-797
Nanobodies as therapeutics: big opportunities for small antibodies.
Drug Discov. Today. 2016; 21: 1076-1113
Thermal unfolding of a llama antibody fragment: a two-state reversible process.
Biochemistry. 2001; 40: 74-83
Potent enzyme inhibitors derived from dromedary heavy-chain antibodies.
EMBO J. 1998; 17: 3512-3520
Single-domain antibody fragments with high conformational stability.
Protein Sci. Publ. Protein Soc. 2002; 11: 500-515
Engineered single-domain antibodies with high protease resistance and thermal stability.
PLoS One. 2011; 6e28218
Escherichia coli F4 fimbriae specific llama single-domain antibody fragments effectively inhibit bacterial adhesion in vitro but poorly protect against diarrhoea.
Vet. Microbiol. 2005; 111: 89-98
Nanobody mediated inhibition of attachment of F18 fimbriae expressing Escherichia coli.
PLoS One. 2014; 9e114691
Enhancement of toxin- and virus-neutralizing capacity of single-domain antibody fragments by N-glycosylation.
Appl. Microbiol. Biotechnol. 2009; 84: 1087-1094
Orally fed seeds producing designer IgAs protect weaned piglets against enterotoxigenic Escherichia coli infection.
Proc. Natl. Acad. Sci. U. S. A. 2013; 110: 11809-11814
Yeast-secreted, dried and food-admixed monomeric IgA prevents gastrointestinal infection in a piglet model.
Nat. Biotechnol. 2019; 37: 527-530
Orally active bivalent VHH construct prevents proliferation of F4+ enterotoxigenic Escherichia coli in weaned piglets.
iScience. 2022; 25104003
Nanobodies targeting conserved epitopes on the major outer membrane protein of Campylobacter as potential tools for control of Campylobacter colonization.
Vet. Res. 2017; 48: 86
In planta expression of nanobody-based designer chicken antibodies targeting Campylobacter.
PLoS One. 2018; 13e0204222
Pentavalent single-domain antibodies reduce Campylobacter jejuni motility and colonization in chickens.
PLoS One. 2013; 8e83928
Protease-resistant single-domain antibodies inhibit Campylobacter jejuni motility.
Protein Eng. Des. Sel. 2014; 27: 191-198
The different ecological niches of enterotoxigenic Escherichia coli.
Environ. Microbiol. 2016; 18: 741-751
Escherichia coli in postweaning diarrhea in pigs: an update on bacterial types, pathogenesis, and prevention strategies.
Anim. Health Res. Rev. 2005; 6: 17-39
Prevalence of virulence factors in enterotoxigenic Escherichia coli isolated from pigs with post-weaning diarrhoea in Europe.
Porc. Health Manag. 2016; 2: 20
Secretory component delays the conversion of secretory IgA into antigen-binding competent F(ab′)2: a possible implication for mucosal defense.
J. Immunol. 1998; 161: 5445-5453
Global epidemiology of Campylobacter infection.
Clin. Microbiol. Rev. 2015; 28: 687-720
Ghahroudi, M.A. et al. National Research Council of Canada. Anti-Campylobacter jejuni antibodies and uses therefor, US9926363B2
Abnousi, H. et al. Novobind Livestock Therapeutics Inc. Antibodies against aquaculture disease-causing agents and uses thereof, WO2020008254A1
Abnousi, H. et al. Novobind Livestock Therapeutics Inc. Antibodies against disease causing agents of poultry and uses thereof, WO2020234642A1
Laustsen, A.H. et al. Bactolife Aps. Pathogen binding proteins, US20220119505A1
Bovine colostrum and its potential for human health and nutrition.
Front. Nutr. 2021; 8651721
Production of bovine colostrum for human consumption to improve health.
Front. Pharmacol. 2022; 12796824
Bovine colostrum: its constituents and uses.
Nutrients. 2021; 13: 265
Global burden of bacterial antimicrobial resistance in 2019: a systematic analysis.
Lancet. 2022; 399: 629-655
WHO preferred product characteristics for vaccines against enterotoxigenic Escherichia coli.
World Health Organization, 2021
Anti-CfaE nanobodies provide broad cross-protection against major pathogenic enterotoxigenic Escherichia coli strains, with implications for vaccine design.
Sci. Rep. 2021; 11: 2751
A nanobody targeting the translocated intimin receptor inhibits the attachment of enterohemorrhagic E. coli to human colonic mucosa.
PLoS Pathog. 2019; 15e1008031
Nanobody-based bispecific neutralizer for Shiga toxin-producing E. coli.
ACS Infect. Dis. 2022; 8: 321-329
Recombinant monovalent llama-derived antibody fragments (VHH) to rotavirus VP6 protect neonatal gnotobiotic piglets against human rotavirus-induced diarrhea.
PLoS Pathog. 2013; 9e1003334
Anti-rotavirus protein reduces stool output in infants with diarrhea: a randomized placebo-controlled trial.
Gastroenterology. 2013; 145: 740-748
Anti-VP6 VHH: an experimental treatment for rotavirus A-associated disease.
PLoS One. 2016; 11e0162351
Selection of nanobodies that block the enzymatic and cytotoxic activities of the binary Clostridium difficile toxin CDT.
Sci. Rep. 2015; 5: 7850
A tetraspecific VHH-based neutralizing antibody modifies disease outcome in three animal models of Clostridium difficile infection.
Clin. Vaccine Immunol. 2016; 23: 774-784
A camelid single-domain antibody neutralizes botulinum neurotoxin A by blocking host receptor binding.
Sci. Rep. 2017; 7: 7438
Bacterial cell factories for recombinant protein production; expanding the catalogue.
Microb. Cell Factories. 2013; 12: 113
Lactic acid bacteria as mucosal delivery vehicles: a realistic therapeutic option.
Appl. Microbiol. Biotechnol. 2016; 100: 5691-5701
Lactic acid bacteria: reviewing the potential of a promising delivery live vector for biomedical purposes.
Microb. Cell Factories. 2015; 14: 137
Co-expression of anti-rotavirus proteins (llama VHH antibody fragments) in Lactobacillus: development and functionality of vectors containing two expression cassettes in tandem.
PLoS One. 2014; 9e96409
Recombinant Limosilactobacillus (Lactobacillus) delivering nanobodies against Clostridium perfringens NetB and alpha toxin confers potential protection from necrotic enteritis.
MicrobiologyOpen. 2022; 11e1270
Neutralization of Clostridium difficile toxin B mediated by engineered Lactobacilli that produce single-domain antibodies.
Infect. Immun. 2016; 84: 395-406
An exopolysaccharide-deficient mutant of Lactobacillus rhamnosus GG efficiently displays a protective llama antibody fragment against rotavirus on its surface.
Appl. Environ. Microbiol. 2015; 81: 5784-5793
Expression of single-domain antibody in different systems.
Appl. Microbiol. Biotechnol. 2018; 102: 539-551
Antibody expressing pea seeds as fodder for prevention of gastrointestinal parasitic infections in chickens.
BMC Biotechnol. 2009; 9: 79
Development of spirulina for the manufacture and oral delivery of protein therapeutics.
Nat. Biotechnol. 2022; 40: 956-964
Plant-produced chimeric VHH–sIgA against enterohemorrhagic E. coli intimin shows cross-serotype inhibition of bacterial adhesion to epithelial cells.
Front. Plant Sci. 2019; 10: 270
Plant molecular farming: a viable platform for recombinant biopharmaceutical production.
Plants. 2020; 9: 842
Comparison of VHH-Fc antibody production in Arabidopsis thaliana, Nicotiana benthamiana and Pichia pastoris.
Plant Biotechnol. J. 2015; 13: 938-947
Improved performance of Eimeria-infected chickens fed corn expressing a single-domain antibody against interleukin-10.
Nat. Food. 2020; 1: 119-126
Rice-based oral antibody fragment prophylaxis and therapy against rotavirus infection.
J. Clin. Invest. 2013; 123: 3829-3838
Development of antibody-fragment-producing rice for neutralization of human norovirus.
Front. Plant Sci. 2021; 12639953
Therapeutic recombinant protein production in plants: challenges and opportunities.
Plants People Planet. 2020; 2: 121-132
Principles of N-linked glycosylation variations of IgG-based therapeutics: pharmacokinetic and functional considerations.
Antibodies. 2020; 9: 22
Highly efficient production of VHH antibody fragments in Brevibacillus choshinensis expression system.
Protein Expr. Purif. 2015; 105: 23-32
A guide to: generation and design of nanobodies.
FEBS J. 2021; 288: 2084-2102
Advances in antibody phage display technology.
Drug Discov. Today. 2022; 27: 2151-2169
A bacterial-two-hybrid selection system for one-step isolation of intracellularly functional nanobodies.
Arch. Biochem. Biophys. 2012; 526: 114-123
Nanobody stability engineering by employing the ΔTm shift; a comparison with apparent rate constants of heat-induced aggregation.
Protein Eng. Des. Sel. 2019; 32: 241-249
Stabilization of an immunoglobulin fold domain by an engineered disulfide bond at the buried hydrophobic region.
J. Biol. Chem. 2007; 282: 36489-36495
Disulfide bond introduction for general stabilization of immunoglobulin heavy-chain variable domains.
J. Mol. Biol. 2008; 377: 478-488
The simulator of the human intestinal microbial ecosystem (SHIME®).
in: Verhoeckx K. The Impact of Food Bioactives on Health: In Vitro and Ex Vivo Models. Springer, 2015: 305-317
The TNO gastro-intestinal model (TIM).
in: Verhoeckx K. The Impact of Food Bioactives on Health: In Vitro and Ex Vivo Models. Springer Open, 2015: 37-46
INFOGEST static in vitro simulation of gastrointestinal food digestion.
Nat. Protoc. 2019; 14: 991-1014
Gastrointestinal function.
in: Kaneko J. Clinical Biochemistry of Domestic Animals. 6th edn. 2008: 413-457
Salivary pH: a diagnostic biomarker.
J. Indian Soc. Periodontol. 2013; 17: 461-465
Estimating drug solubility in the gastrointestinal tract.
Adv. Drug Deliv. Rev. 2007; 59: 591-602
A general approach for stabilizing nanobodies for intracellular expression.
eLife. 2022; 11e68253
Preparation of a nanobody specific to dectin 1 and its anti-inflammatory effects on fungal keratitis.
Int. J. Nanomedicine. 2022; 17: 537-551
Understanding the mechanisms and drivers of antimicrobial resistance.
Lancet. 2016; 387: 176-187
Distinct increase in antimicrobial resistance genes among Escherichia coli during 50 years of antimicrobial use in livestock production in China.
Nat. Food. 2022; 3: 197-205
Molecular mechanisms of antibiotic resistance.
Chem. Commun. 2011; 47: 4055-4061
Strategies to combat antimicrobial resistance.
J. Clin. Diagn. Res. 2014; 8: ME01-ME04
Evolution of bacterial virulence.
FEMS Microbiol. Rev. 2017; 41: 679-697
Regulation of virulence: the rise and fall of gastrointestinal pathogens.
J. Gastroenterol. 2016; 51: 195-205
Virulence factors of enteric pathogenic Escherichia coli: a review.
Int. J. Mol. Sci. 2021; 22: 9922
Pathogenic Escherichia coli.
Nat. Rev. Microbiol. 2004; 2: 123-140
Anti-virulence strategies to combat bacteria-mediated disease.
Nat. Rev. Drug Discov. 2010; 9: 117-128
- SEO Powered Content & PR Distribution. Get Amplified Today.
- Platoblockchain. Web3 Metaverse Intelligence. Knowledge Amplified. Access Here.
- Source: https://www.cell.com/trends/biotechnology/fulltext/S0167-7799(23)00033-1?rss=yes
- 1
- 2019
- 50 Years
- a
- Action
- active
- activities
- against
- agents
- aggregation
- Alpha
- among
- analysis
- and
- animal
- animals
- Antibodies
- apparent
- approach
- authors
- Bacteria
- Big
- binding
- biomarker
- biomedical
- Block
- blocking
- bond
- broad
- burden
- Canada
- Capacity
- causing
- Cells
- challenges
- characteristics
- China
- Clinical
- combat
- comparison
- competent
- component
- considerations
- construct
- consumption
- control
- Conversion
- Council
- Defense
- delays
- delivered
- delivering
- delivery
- Derived
- Design
- Designer
- Development
- different
- discovery
- Disease
- Display
- displays
- domain
- domains
- Domestic
- drivers
- drug
- during
- Ecological
- ecosystem
- effectively
- effects
- efficient
- efficiently
- Engineering
- Europe
- expanding
- factories
- factors
- Fall
- farming
- Fed
- food
- from
- function
- functional
- functionality
- General
- generation
- guide
- Health
- High
- host
- HTTPS
- human
- Impact
- implications
- improve
- in
- Inc.
- Increase
- Indian
- infection
- Infections
- Introduction
- Invest
- isolated
- isolation
- learning
- List
- live
- Llama
- machine
- machine learning
- major
- mechanism
- Meyer
- Milk
- model
- models
- MOL
- molecular
- moving
- Nanomedicine
- National
- Natural
- nutrition
- ONE
- opportunities
- Option
- organization
- Outcome
- People
- performance
- plan
- planet
- plants
- platform
- plato
- Plato Data Intelligence
- PlatoData
- possible
- potential
- Poultry
- preferred
- Prevention
- process
- produce
- Product
- Production
- Profile
- promising
- protect
- protection
- Protective
- Protein
- Proteins
- provide
- purposes
- Randomized
- Rate
- realistic
- recognition
- reduce
- reduces
- region
- research
- Resistance
- review
- reviewing
- Rice
- Rise
- Rival
- SCI
- seeds
- selection
- shift
- Shows
- significance
- simulation
- simulator
- single
- small
- specific
- Stability
- Strains
- strategies
- Surface
- system
- Systems
- Tandem
- targeting
- Technologies
- Technology
- The
- Therapeutic
- therapeutics
- therapy
- thermal
- three
- Tim
- to
- today
- tools
- top
- treatment
- trial
- types
- unfolding
- Update
- use
- Vaccine
- vaccines
- Vehicles
- viable
- vivo
- W
- X
- years
- zephyrnet