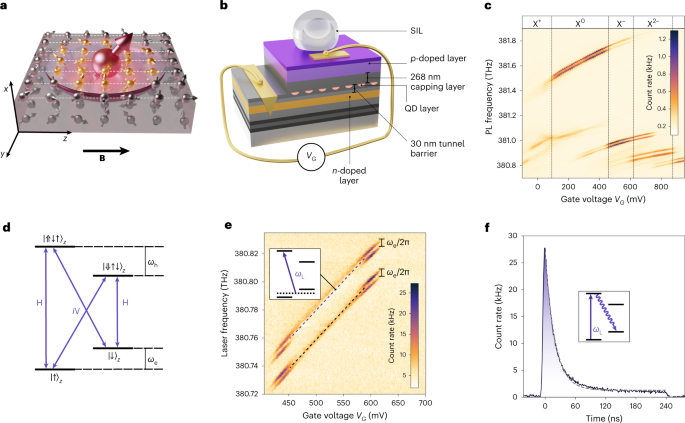
Nickerson, N. H., Fitzsimons, J. F. & Benjamin, S. C. Freely scalable quantum technologies using cells of 5-to-50 qubits with very lossy and noisy photonic links. Phys. Rev. X 4, 041041 (2014).
Monroe, C. et al. Large-scale modular quantum-computer architecture with atomic memory and photonic interconnects. Phys. Rev. A 89, 022317 (2014).
Cohen, L. Z., Kim, I. H., Bartlett, S. D. & Brown, B. J. Low-overhead fault-tolerant quantum computing using long-range connectivity. Sci. Adv. 8, eabn1717 (2022).
Gimeno-Segovia, M., Shadbolt, P., Browne, D. E. & Rudolph, T. From three-photon Greenberger-Horne-Zeilinger states to ballistic universal quantum computation. Phys. Rev. Lett. 115, 020502 (2015).
Stephenson, L. J. et al. High-rate, high-fidelity entanglement of qubits across an elementary quantum network. Phys. Rev. Lett. 124, 110501 (2020).
Postler, L. et al. Demonstration of fault-tolerant universal quantum gate operations. Nature 605, 675–680 (2022).
Abobeih, M. H. et al. Fault-tolerant operation of a logical qubit in a diamond quantum processor. Nature 606, 884–889 (2022).
Bergeron, L. et al. Silicon-integrated telecommunications photon-spin interface. PRX Quantum 1, 020301 (2020).
Christle, D. J. et al. Isolated spin qubits in SiC with a high-fidelity infrared spin-to-photon interface. Phys. Rev. X 7, 021046 (2017).
Ruskuc, A., Wu, C.-J., Rochman, J., Choi, J. & Faraon, A. Nuclear spin-wave quantum register for a solid-state qubit. Nature 602, 408–413 (2022).
Raha, M. et al. Optical quantum nondemolition measurement of a single rare earth ion qubit. Nat. Commun. 11, 1605 (2020).
Berezovsky, J., Mikkelsen, M. H., Stoltz, N. G., Coldren, L. A. & Awschalom, D. D. Picosecond coherent optical manipulation of a single electron spin in a quantum dot. Science 320, 349–352 (2008).
De Greve, K. et al. Ultrafast coherent control and suppressed nuclear feedback of a single quantum dot hole qubit. Nat. Phys. 7, 872–878 (2011).
Godden, T. M. et al. Coherent optical control of the spin of a single hole in an InAs/GaAs quantum dot. Phys. Rev. Lett. 108, 017402 (2012).
Pfaff, W. et al. Unconditional quantum teleportation between distant solid-state quantum bits. Science 345, 532–535 (2014).
Pompili, M. et al. Realization of a multinode quantum network of remote solid-state qubits. Science 372, 259–264 (2021).
Schwartz, I. et al. Deterministic generation of a cluster state of entangled photons. Science 354, 434–437 (2016).
Istrati, D. et al. Sequential generation of linear cluster states from a single photon emitter. Nat. Commun. 11, 5501 (2020).
Wang, H. et al. Towards optimal single-photon sources from polarized microcavities. Nat. Photon. 13, 770–775 (2019).
Liu, J. et al. A solid-state source of strongly entangled photon pairs with high brightness and indistinguishability. Nat. Nanotechnol. 14, 586–593 (2019).
Tomm, N. et al. A bright and fast source of coherent single photons. Nat. Nanotechnol. 16, 399–403 (2021).
Appel, M. H. et al. Coherent spin-photon interface with waveguide induced cycling transitions. Phys. Rev. Lett. 126, 013602 (2021).
Thomas, S. E. et al. Bright polarized single-photon source based on a linear dipole. Phys. Rev. Lett. 126, 233601 (2021).
Varnava, M., Browne, D. E. & Rudolph, T. How good must single photon sources and detectors be for efficient linear optical quantum computation? Phys. Rev. Lett. 100, 060502 (2008).
Pant, M., Towsley, D., Englund, D. & Guha, S. Percolation thresholds for photonic quantum computing. Nat. Commun. 10, 1070 (2019).
Press, D., Ladd, T. D., Zhang, B. & Yamamoto, Y. Complete quantum control of a single quantum dot spin using ultrafast optical pulses. Nature 456, 218–221 (2008).
De Greve, K. et al. Quantum-dot spin–photon entanglement via frequency downconversion to telecom wavelength. Nature 491, 421–425 (2012).
Appel, M. H. et al. Entangling a hole spin with a time-bin photon: a waveguide approach for quantum dot sources of multiphoton entanglement. Phys. Rev. Lett. 128, 233602 (2022).
Delteil, A. et al. Generation of heralded entanglement between distant hole spins. Nat. Phys. 12, 218–223 (2016).
Stockill, R. et al. Phase-tuned entangled state generation between distant spin qubits. Phys. Rev. Lett. 119, 010503 (2017).
Gangloff, D. A. et al. Quantum interface of an electron and a nuclear ensemble. Science 364, 62–66 (2019).
Taylor, J. M., Marcus, C. M. & Lukin, M. D. Long-lived memory for mesoscopic quantum bits. Phys. Rev. Lett. 90, 206803 (2003).
Denning, E. V., Gangloff, D. A., Atatüre, M., Mørk, J. & Le Gall, C. Collective quantum memory activated by a driven central spin. Phys. Rev. Lett. 123, 140502 (2019).
Stockill, R. et al. Quantum dot spin coherence governed by a strained nuclear environment. Nat. Commun. 7, 12745 (2016).
Gong, Q., Offermans, P., Nötzel, R., Koenraad, P. M. & Wolter, J. H. Capping process of InAs/GaAs quantum dots studied by cross-sectional scanning tunneling microscopy. Appl. Phys. Lett. 85, 5697 (2004).
Bechtold, A. et al. Three-stage decoherence dynamics of an electron spin qubit in an optically active quantum dot. Nat. Phys. 11, 1005–1008 (2015).
Covre da Silva, S. F. et al. GaAs quantum dots grown by droplet etching epitaxy as quantum light sources. Appl. Phys. Lett. 119, 120502 (2021).
Schöll, E. et al. Resonance fluorescence of GaAs quantum dots with near-unity photon indistinguishability. Nano Lett. 19, 2404–2410 (2019).
Zhai, L. et al. Low-noise GaAs quantum dots for quantum photonics. Nat. Commun. 11, 4745 (2020).
Chekhovich, E. A., Covre da Silva, S. F. & Rastelli, A. Nuclear spin quantum register in an optically active semiconductor quantum dot. Nat. Nanotechnol. 15, 999–1004 (2020).
Schimpf, C., Manna, S., Covre da Silva, S. F., Aigner, M. & Rastelli, A. Entanglement-based quantum key distribution with a blinking-free quantum dot operated at a temperature up to 20 K. Adv. Photon. 3, 065001 (2021).
Kuhlmann, A. V. et al. Charge noise and spin noise in a semiconductor quantum device. Nat. Phys. 9, 570–575 (2013).
Gillard, G. et al. Fundamental limits of electron and nuclear spin qubit lifetimes in an isolated self-assembled quantum dot. npj Quantum Inf. 7, 43 (2021).
Bodey, J. H. et al. Optical spin locking of a solid-state qubit. npj Quantum Inf. 5, 95 (2019).
Cywiński, Ł., Witzel, W. M. & Das Sarma, S. Pure quantum dephasing of a solid-state electron spin qubit in a large nuclear spin bath coupled by long-range hyperfine-mediated interactions. Phys. Rev. B 79, 245314 (2009).
Botzem, T. et al. Quadrupolar and anisotropy effects on dephasing in two-electron spin qubits in GaAs. Nat. Commun. 7, 11170 (2016).
Bluhm, H. et al. Dephasing time of GaAs electron-spin qubits coupled to a nuclear bath exceeding 200 μs. Nat. Phys. 7, 109–113 (2011).
Malinowski, F. K. et al. Notch filtering the nuclear environment of a spin qubit. Nat. Nanotechnol. 12, 16–20 (2016).
Malinowski, F. K. et al. Spectrum of the nuclear environment for GaAs spin qubits. Phys. Rev. Lett. 118, 177702 (2017).
de Lange, G., Wang, Z. H., Ristè, D., Dobrovitski, V. V. & Hanson, R. Universal dynamical decoupling of a single solid-state spin from a spin bath. Science 330, 60–63 (2010).
Huthmacher, L. et al. Coherence of a dynamically decoupled quantum-dot hole spin. Phys. Rev. B 97, 241413 (2018).
Ragunathan, G. Nuclear Spin Phenomena in III-V and II-VI Semiconductor Quantum Dots. PhD thesis, University of Sheffield (2019).
Ulhaq, A. et al. Vanishing electron g factor and long-lived nuclear spin polarization in weakly strained nanohole-filled GaAs/AlGaAs quantum dots. Phys. Rev. B 93, 165306 (2016).
Chekhovich, E. A., Hopkinson, M., Skolnick, M. S. & Tartakovskii, A. I. Suppression of nuclear spin bath fluctuations in self-assembled quantum dots induced by inhomogeneous strain. Nat. Commun. 6, 6348 (2015).
Knijn, P. J. et al. A solid-state NMR and DFT study of compositional modulations in AlxGa1−xAs. Phys. Chem. Chem. Phys. 12, 11517–11535 (2010).
Chekhovich, E. A. et al. Measurement of the spin temperature of optically cooled nuclei and GaAs hyperfine constants in GaAs/AlGaAs quantum dots. Nat. Mater. 16, 982–986 (2017).
van Bree, J. et al. Anisotropy of electron and hole g tensors of quantum dots: an intuitive picture based on spin-correlated orbital currents. Phys. Rev. B 93, 035311 (2016).
Wolters, J. et al. Simple atomic quantum memory suitable for semiconductor quantum dot single photons. Phys. Rev. Lett. 119, 060502 (2017).
Heyn, C. et al. Highly uniform and strain-free GaAs quantum dots fabricated by filling of self-assembled nanoholes. Appl. Phys. Lett. 94, 183113 (2009).
Atkinson, P., Zallo, E. & Schmidt, O. G. Independent wavelength and density control of uniform GaAs/AlGaAs quantum dots grown by infilling self-assembled nanoholes. J. Appl. Phys. 112, 054303 (2012).
Huo, Y. H., Rastelli, A. & Schmidt, O. G. Ultra-small excitonic fine structure splitting in highly symmetric quantum dots on GaAs (001) substrate. Appl. Phys. Lett. 102, 152105 (2013).
Houel, J. et al. Probing single-charge fluctuations at a GaAs/AlAs interface using laser spectroscopy on a nearby InGaAs quantum dot. Phys. Rev. Lett. 108, 107401 (2012).
Virtanen, P. et al. SciPy 1.0: fundamental algorithms for scientific computing in Python. Nat. Methods 17, 261–272 (2020).
Chekhovich, E. A. et al. Cross calibration of deformation potentials and gradient-elastic tensors of GaAs using photoluminescence and nuclear magnetic resonance spectroscopy in GaAs/AlGaAs quantum dot structures. Phys. Rev. B 97, 235311 (2018).
Neder, I. et al. Semiclassical model for the dephasing of a two-electron spin qubit coupled to a coherently evolving nuclear spin bath. Phys. Rev. B 84, 035441 (2011).
- SEO Powered Content & PR Distribution. Get Amplified Today.
- Platoblockchain. Web3 Metaverse Intelligence. Knowledge Amplified. Access Here.
- Source: https://www.nature.com/articles/s41565-022-01282-2
- 1
- 10
- 100
- 11
- 2011
- 2012
- 2014
- 2016
- 2017
- 2018
- 2019
- 2020
- 2021
- 2022
- 28
- 39
- 7
- 84
- 9
- a
- across
- active
- algorithms
- and
- approach
- architecture
- article
- based
- Benjamin
- between
- Cells
- central
- charge
- Cluster
- COHERENT
- Collective
- complete
- computation
- computing
- Connectivity
- control
- coupled
- Cross
- DA
- device
- Diamond
- Distant
- distribution
- DOT
- driven
- dynamics
- earth
- effects
- efficient
- Environment
- Ether (ETH)
- evolving
- FAST
- feedback
- filtering
- fine
- fluctuations
- Frequency
- from
- fundamental
- generation
- good
- grown
- High
- highly
- Hole
- How
- HTTPS
- ideal
- in
- independent
- interactions
- Interface
- intuitive
- isolated
- Key
- Kim
- large
- large-scale
- laser
- light
- limits
- LINK
- links
- Manipulation
- Marcus
- Memory
- Microscopy
- model
- modular
- multiphoton
- Nature
- network
- Noise
- nuclear
- operated
- operation
- Operations
- optimal
- pairs
- Photons
- picture
- plato
- Plato Data Intelligence
- PlatoData
- process
- Processor
- Python
- Quantum
- quantum computing
- Quantum dot
- Quantum dots
- quantum gate
- Qubit
- qubits
- RARE
- realization
- register
- remote
- resonance
- scalable
- scanning
- semiconductor
- Simple
- single
- Source
- Sources
- Spectroscopy
- Spectrum
- Spin
- spin qubits
- spins
- State
- States
- strong
- strongly
- structure
- studied
- Study
- suitable
- suppression
- Technologies
- telecom
- telecommunications
- The
- time
- to
- towards
- transitions
- unconditional
- under
- Universal
- university
- via
- W
- wu
- zephyrnet