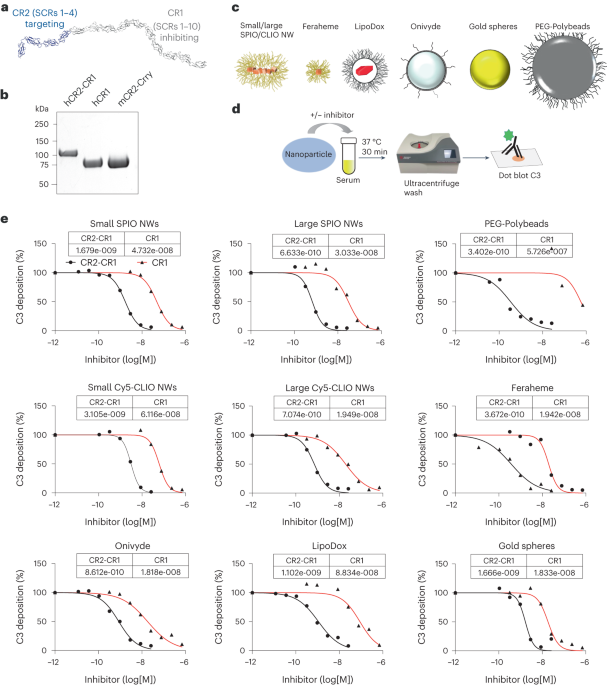
La-Beck, N. M., Islam, M. R. & Markiewski, M. M. Nanoparticle-induced complement activation: implications for cancer nanomedicine. Front. Immunol. 11, 603039 (2020).
Moghimi, S. M., Simberg, D., Papini, E. & Farhangrazi, Z. S. Complement activation by drug carriers and particulate pharmaceuticals: principles, challenges and opportunities. Adv. Drug Deliv. Rev. 157, 83–95 (2020).
Ricklin, D., Hajishengallis, G., Yang, K. & Lambris, J. D. Complement: a key system for immune surveillance and homeostasis. Nat. Immunol. 11, 785–797 (2010).
Szebeni, J., Simberg, D., Gonzalez-Fernandez, A., Barenholz, Y. & Dobrovolskaia, M. A. Roadmap and strategy for overcoming infusion reactions to nanomedicines. Nat. Nanotechnol. 13, 1100–1108 (2018).
Moghimi, S. M. et al. Material properties in complement activation. Adv. Drug Deliv. Rev. 63, 1000–1007 (2011).
Tavano, R. et al. C1q-mediated complement activation and C3 opsonization trigger recognition of stealth poly(2-methyl-2-oxazoline)-coated cilica nanoparticles by human phagocytes. ACS Nano 12, 5834–5847 (2018).
Inturi, S. et al. Modulatory role of surface coating of superparamagnetic iron oxide nanoworms in complement opsonization and leukocyte pptake. ACS Nano 9, 10758–10768 (2015).
Dobrovolskaia, M. A., Aggarwal, P., Hall, J. B. & McNeil, S. E. Preclinical studies to understand nanoparticle interaction with the immune system and its potential effects on nanoparticle biodistribution. Mol. Pharm. 5, 487–495 (2008).
Ricklin, D. & Lambris, J. D. Complement in immune and inflammatory disorders: pathophysiological mechanisms. J. Immunol. 190, 3831–3838 (2013).
Moghimi, S. M. Cancer nanomedicine and the complement system activation paradigm: anaphylaxis and tumour growth. J. Control. Release 190, 556–562 (2014).
Forneris, F. et al. Regulators of complement activity mediate inhibitory mechanisms through a common C3b-binding mode. EMBO J. 35, 1133–1149 (2016).
Schmidt, C. Q., Lambris, J. D. & Ricklin, D. Protection of host cells by complement regulators. Immunol. Rev. 274, 152–171 (2016).
Zipfel, P. F. & Skerka, C. Complement regulators and inhibitory proteins. Nat. Rev. Immunol. 9, 729–740 (2009).
Morgan, B. P. & Harris, C. L. Complement, a target for therapy in inflammatory and degenerative diseases. Nat. Rev. Drug Discov. 14, 857–877 (2015).
Mastellos, D. C., Ricklin, D. & Lambris, J. D. Clinical promise of next-generation complement therapeutics. Nat. Rev. Drug Discov. 18, 707–729 (2019).
Smith, G. P. & Smith, R. A. Membrane-targeted complement inhibitors. Mol. Immunol. 38, 249–255 (2001).
Gaikwad, H. et al. Complement inhibitors block complement C3 opsonization and improve targeting selectivity of nanoparticles in blood. Bioconjugate Chem. 31, 1844–1856 (2020).
Gifford, G. et al. Complement therapeutics meets nanomedicine: overcoming human complement activation and leukocyte uptake of nanomedicines with soluble domains of CD55. J. Control. Release 302, 181–189 (2019).
Belling, J. N. et al. Stealth immune properties of graphene oxide enabled by surface-bound complement factor H. ACS Nano 10, 10161–10172 (2016).
Wang, Z. et al. Combating complement’s deleterious effects on nanomedicine by conjugating complement regulatory proteins to nanoparticles. Adv. Mater. 34, e2107070 (2022).
Souza, D. G., Esser, D., Bradford, R., Vieira, A. T. & Teixeira, M. M. APT070 (Mirococept), a membrane-localised complement inhibitor, inhibits inflammatory responses that follow intestinal ischaemia and reperfusion injury. Br. J. Pharmacol. 145, 1027–1034 (2005).
White, J. et al. Biological activity, membrane-targeting modification, and crystallization of soluble human decay accelerating factor expressed in E. coli. Protein Sci. 13, 2406–2415 (2004).
Bechtler, C. et al. Complement-regulatory biomaterial coatings: activity and selectivity profile of the factor H-binding peptide 5C6. Acta Biomater. 155, 123–138 (2023).
Hare, J. I. et al. Challenges and strategies in anti-cancer nanomedicine development: an industry perspective. Adv. Drug Deliv. Rev. 108, 25–38 (2017).
Benamu, E. & Montoya, J. G. Infections associated with the use of eculizumab: recommendations for prevention and prophylaxis. Curr. Opin. Infect. Dis. 29, 319–329 (2016).
Barnum, S. R. Therapeutic inhibition of complement: well worth the risk. Trend Pharmacol. Sci. 38, 503–505 (2017).
van den Elsen, J. M. & Isenman, D. E. A crystal structure of the complex between human complement receptor 2 and its ligand C3d. Science 332, 608–611 (2011).
Farries, T. C., Seya, T., Harrison, R. A. & Atkinson, J. P. Competition for binding sites on C3b by CR1, CR2, MCP, factor B and factor H. Complement Inflamm. 7, 30–41 (1990).
Holers, M. et al. The human complement receptor type 2 (CR2)/CR1 fusion protein TT32, a targeted inhibitor of the classical and alternative pathway C3 convertases, prevents arthritis in active immunization and passive transfer models and acts by CR2-dependent targeting of CR1 regulatory activity. Immunobiology 217, 1210–1210 (2012).
Holers, V. M., Rohrer, B. & Tomlinson, S. CR2-mediated targeting of complement inhibitors: bench-to-bedside using a novel strategy for site-specific complement modulation. Adv. Exp. Med. Biol. 735, 137–154 (2013).
Huang, Y. X., Qiao, F., Atkinson, C., Holers, V. M. & Tomlinson, S. A novel targeted inhibitor of the alternative pathway of complement and its therapeutic application in ischemia/reperfusion injury. J. Immunol. 181, 8068–8076 (2008).
Risitano, A. M. et al. The complement receptor 2/factor H fusion protein TT30 protects paroxysmal nocturnal hemoglobinuria erythrocytes from complement-mediated hemolysis and C3 fragment. Blood 119, 6307–6316 (2012).
Tomlinson, S. & Thurman, J. M. Tissue-targeted complement therapeutics. Mol. Immunol. 102, 120–128 (2018).
Risitano, A. M. et al. Safety and pharmacokinetics of the complement inhibitor TT30 in a phase I trial for untreated PNH patients. Blood 126, 2137 (2015).
Fridkis-Hareli, M. et al. The human complement receptor type 2 (CR2)/CR1 fusion protein TT32, a novel targeted inhibitor of the classical and alternative pathway C3 convertases, prevents arthritis in active immunization and passive transfer mouse models. Mol. Immunol. 105, 150–164 (2019).
Song, H., Qiao, F., Atkinson, C., Holers, V. M. & Tomlinson, S. A complement C3 inhibitor specifically targeted to sites of complement activation effectively ameliorates collagen-induced arthritis in DBA/1J mice. J. Immunol. 179, 7860–7867 (2007).
Kim, Y. U. et al. Mouse complement regulatory protein Crry/p65 uses the specific mechanisms of both human decay-accelerating factor and membrane cofactor protein. J. Exp. Med. 181, 151–159 (1995).
Benasutti, H. et al. Variability of complement response toward preclinical and clinical nanocarriers in the general population. Bioconjugate Chem. 28, 2747–2755 (2017).
Berger, N. et al. New analogs of the complement C3 inhibitor compstatin with increased solubility and improved pharmacokinetic profile. J. Med. Chem. 61, 6153–6162 (2018).
Lamers, C. et al. Insight into mode-of-action and structural determinants of the compstatin family of clinical complement inhibitors. Nat. Commun. 13, 5519 (2022).
Guthridge, J. M. et al. Epitope mapping using the X-ray crystallographic structure of complement receptor type 2 (CR2)/CD21: identification of a highly inhibitory monoclonal antibody that directly recognizes the CR2-C3d interface. J. Immunol. 167, 5758–5766 (2001).
Chen, F. et al. Complement proteins bind to nanoparticle protein corona and undergo dynamic exchange in vivo. Nat. Nanotechnol. 12, 387–393 (2017).
Venkatesh, Y. P., Minich, T. M., Law, S. K. & Levine, R. P. Natural release of covalently bound C3b from cell surfaces and the study of this phenomenon in the fluid-phase system. J. Immunol. 132, 1435–1439 (1984).
Li, Y. et al. Complement opsonization of nanoparticles: differences between humans and preclinical species. J. Control. Release 338, 548–556 (2021).
Hardy, M. P., Rowe, T. & Wymann, S. Soluble complement receptor 1 therapeutics. J. Immunol. Sci. 6, 1–17 (2022).
Voorhees, A. B., Baker, H. J. & Pulaski, E. J. Reactions of albino rats to injections of dextran. Proc. Soc. Exp. Biol. Med. 76, 254–256 (1951).
Dezsi, L. et al. Complement activation-related pathophysiological changes in anesthetized rats: activator-dependent variations of symptoms and mediators of pseudoallergy. Molecules 24, 3283 (2019).
Timotius, I. K. et al. Combination of defined catwalk gait parameters for predictive locomotion recovery in experimental spinal cord injury rat models. eNeuro 8, 0497-20.2021 (2021).
Chen, E. et al. Premature drug release from polyethylene glycol (PEG)-coated liposomal doxorubicin via formation of the membrane attack complex. ACS Nano 14, 7808–7822 (2020).
Munter, R. et al. Unravelling heterogeneities in complement and antibody opsonization of individual liposomes as a function of surface architecture. Small 18, e2106529 (2022).
Vu, V. P. et al. Immunoglobulin deposition on biomolecule corona determines complement opsonization efficiency of preclinical and clinical nanoparticles. Nat. Nanotechnol. 14, 260–268 (2019).
Wang, G. et al. In vitro and in vivo differences in murine third complement component (C3) opsonization and macrophage/leukocyte responses to antibody-functionalized iron oxide nanoworms. Front. Immunol. 8, 151 (2017).
Wang, G. et al. Activation of human complement system by dextran-coated iron oxide nanoparticles is not affected by dextran/Fe ratio, hydroxyl modifications, and crosslinking. Front. Immunol. 7, 418 (2016).
Moghimi, S. M. & Simberg, D. Critical issues and pitfalls in serum and plasma handling for complement analysis in nanomedicine and bionanotechnology. Nano Today 44, 101479 (2022).
Wu, L.-P. et al. Dendrimer end-terminal motif-dependent evasion of human complement and complement activation through IgM hitchhiking. Nat. Commun. 12, 4858 (2021).
Wang, G. et al. High-relaxivity superparamagnetic iron oxide nanoworms with decreased immune recognition and long-circulating properties. ACS Nano 8, 12437–12449 (2014).
- SEO Powered Content & PR Distribution. Get Amplified Today.
- PlatoData.Network Vertical Generative Ai. Empower Yourself. Access Here.
- PlatoAiStream. Web3 Intelligence. Knowledge Amplified. Access Here.
- PlatoESG. Carbon, CleanTech, Energy, Environment, Solar, Waste Management. Access Here.
- PlatoHealth. Biotech and Clinical Trials Intelligence. Access Here.
- Source: https://www.nature.com/articles/s41565-023-01514-z
- :is
- :not
- ][p
- 01
- 06
- 08
- 09
- 1
- 10
- 11
- 12
- 13
- 14
- 15%
- 16
- 167
- 17
- 179
- 19
- 1951
- 1995
- 20
- 2001
- 2005
- 2008
- 2010
- 2011
- 2012
- 2013
- 2014
- 2015
- 2016
- 2017
- 2018
- 2019
- 2020
- 2021
- 2022
- 2023
- 22
- 23
- 24
- 25
- 26
- 27
- 28
- 29
- 30
- 31
- 32
- 33
- 35%
- 36
- 39
- 40
- 41
- 46
- 49
- 50
- 51
- 52
- 53
- 54
- 7
- 75
- 8
- 9
- a
- accelerating
- Activation
- active
- activity
- acts
- Aggarwal
- AL
- alternative
- an
- analysis
- and
- antibody
- Application
- architecture
- article
- AS
- associated
- attack
- b
- baker
- between
- bind
- binding
- Block
- blood
- both
- bound
- by
- Cancer
- carriers
- CATWALK
- cell
- Cells
- challenges
- Changes
- click
- Clinical
- combating
- combination
- Common
- competition
- Complement
- complex
- component
- control
- Corona
- critical
- Crystal
- decreased
- defined
- Den
- determines
- Development
- differences
- directly
- diseases
- disorders
- domains
- drug
- dynamic
- e
- E. coli
- E&T
- effectively
- effects
- efficiency
- enabled
- Ether (ETH)
- exchange
- experimental
- expressed
- factor
- family
- follow
- For
- formation
- from
- function
- fusion
- General
- Graphene
- Growth
- Hall
- Handling
- highly
- homeostasis
- host
- http
- HTTPS
- human
- Humans
- i
- Identification
- immune
- Immune system
- implications
- improve
- improved
- in
- increased
- individual
- industry
- Infections
- inflammatory
- infusion
- insight
- interaction
- Interface
- into
- issues
- ITS
- Key
- Law
- levine
- LINK
- mapping
- material
- MCP
- mechanisms
- Meets
- mice
- Mode
- models
- Modifications
- mouse
- Nanomedicine
- nanotechnology
- Natural
- Nature
- New
- next-generation
- novel
- of
- on
- opportunities
- overcoming
- paradigm
- parameters
- passive
- pathway
- patients
- Peg
- perspective
- pharmaceuticals
- phase
- phenomenon
- Plasma
- plato
- Plato Data Intelligence
- PlatoData
- population
- potential
- preclinical
- predictive
- Premature
- Prevention
- prevents
- principles
- Profile
- promise
- properties
- protection
- Protein
- Proteins
- R
- RAT
- ratio
- reactions
- recognition
- recognizes
- recommendations
- recovery
- reference
- Regulators
- regulatory
- release
- response
- responses
- Risk
- roadmap
- Role
- s
- Safety
- Scholar
- SCI
- Serum
- Sites
- specific
- specifically
- Stealth
- strategies
- Strategy
- structural
- structure
- studies
- Study
- Surface
- surveillance
- Symptoms
- system
- T
- Target
- targeted
- targeting
- that
- The
- Therapeutic
- therapeutics
- therapy
- Third
- this
- Through
- tips
- to
- toward
- towards
- transfer
- trial
- trigger
- type
- undergo
- understand
- uptake
- use
- uses
- using
- variations
- via
- vivo
- WELL
- with
- worth
- X
- x-ray
- zephyrnet