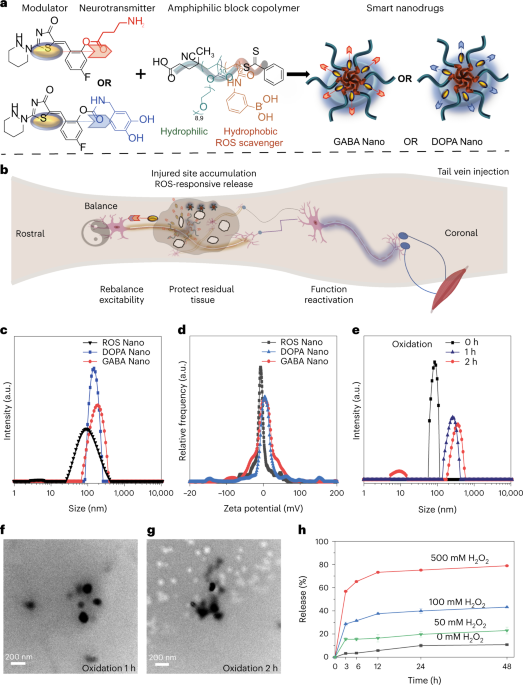
David, S. & Kroner, A. Repertoire of microglial and macrophage responses after spinal cord injury. Nat. Rev. Neurosci. 12, 388–399 (2011).
Block, M. L., Zecca, L. & Hong, J. S. Microglia-mediated neurotoxicity: uncovering the molecular mechanisms. Nat. Rev. Neurosci. 8, 57–69 (2007).
Ulndreaj, A., Badner, A. & Fehlings, M. G. Promising neuroprotective strategies for traumatic spinal cord injury with a focus on the differential effects among anatomical levels of injury. F1000Research 6, 1907 (2017).
Li, L. et al. A MnO2 nanoparticle-dotted hydrogel promotes spinal cord repair via regulating reactive oxygen species microenvironment and synergizing with mesenchymal stem cells. ACS Nano 13, 14283–14293 (2019).
Zhang, N. et al. A 3D fiber-hydrogel based non-viral gene delivery platform reveals that microRNAs promote axon regeneration and enhance functional recovery following spinal cord injury. Adv. Sci. 8, e2100805 (2021).
Chen, B. et al. Reactivation of dormant relay pathways in injured spinal cord by KCC2 manipulations. Cell 174, 521–535.e13 (2018).
Wilson, J. M., Blagovechtchenski, E. & Brownstone, R. M. Genetically defined inhibitory neurons in the mouse spinal cord dorsal horn: a possible source of rhythmic inhibition of motoneurons during fictive locomotion. J. Neurosci. 30, 1137–1148 (2010).
Haring, M. et al. Neuronal atlas of the dorsal horn defines its architecture and links sensory input to transcriptional cell types. Nat. Neurosci. 21, 869–880 (2018).
Brommer, B. et al. Improving hindlimb locomotor function by non-invasive AAV-mediated manipulations of propriospinal neurons in mice with complete spinal cord injury. Nat. Commun. 12, 781 (2021).
Courtine, G. & Sofroniew, M. V. Spinal cord repair: advances in biology and technology. Nat. Med. 25, 898–908 (2019).
Ramirez-Jarquin, U. N., Lazo-Gomez, R., Tovar, Y. R. L. B. & Tapia, R. Spinal inhibitory circuits and their role in motor neuron degeneration. Neuropharmacology 82, 101–107 (2014).
Matsuya, R., Ushiyama, J. & Ushiba, J. Inhibitory interneuron circuits at cortical and spinal levels are associated with individual differences in corticomuscular coherence during isometric voluntary contraction. Sci. Rep. 7, 44417 (2017).
Ramirez-Jarquin, U. N. & Tapia, R. Excitatory and inhibitory neuronal circuits in the spinal cord and their role in the control of motor neuron function and degeneration. ACS Chem. Neurosci. 9, 211–216 (2018).
Rivera, C. et al. The K+/Cl– co-transporter KCC2 renders GABA hyperpolarizing during neuronal maturation. Nature 397, 251–255 (1999).
Boulenguez, P. et al. Down-regulation of the potassium-chloride cotransporter KCC2 contributes to spasticity after spinal cord injury. Nat. Med. 16, 302–307 (2010).
Gagnon, M. et al. Chloride extrusion enhancers as novel therapeutics for neurological diseases. Nat. Med. 19, 1524–1528 (2013).
Reinig, S., Driever, W. & Arrenberg, A. B. The descending diencephalic dopamine system is tuned to sensory stimuli. Curr. Biol. 27, 318–333 (2017).
Li, Y. et al. Pericytes impair capillary blood flow and motor function after chronic spinal cord injury. Nat. Med. 23, 733–741 (2017).
Sharples, S. A. et al. A dynamic role for dopamine receptors in the control of mammalian spinal networks. Sci. Rep. 10, 16429 (2020).
Grillner, S. & Jessell, T. M. Measured motion: searching for simplicity in spinal locomotor networks. Curr. Opin. Neurobiol. 19, 572–586 (2009).
Li, W. C. & Moult, P. R. The control of locomotor frequency by excitation and inhibition. J. Neurosci. 32, 6220–6230 (2012).
Kiehn, O. Decoding the organization of spinal circuits that control locomotion. Nat. Rev. Neurosci. 17, 224–238 (2016).
Jiang, X. C. et al. Neural stem cells transfected with reactive oxygen species–responsive polyplexes for effective treatment of ischemic stroke. Adv. Mater. 31, e1807591 (2019).
Liu, P. et al. Biomimetic dendrimer–peptide conjugates for early multi-target therapy of Alzheimer’s disease by inflammatory microenvironment modulation. Adv. Mater. 33, e2100746 (2021).
Lu, Y. et al. Microenvironment remodeling micelles for Alzheimer’s disease therapy by early modulation of activated microglia. Adv. Sci. 6, 1801586 (2019).
Xu, W. et al. Increased production of reactive oxygen species contributes to motor neuron death in a compression mouse model of spinal cord injury. Spinal Cord 43, 204–213 (2005).
Zhang, M. et al. Oxidation and temperature dual responsive polymers based on phenylboronic acid and N-isopropylacrylamide motifs. Polym. Chem. 7, 1494–1504 (2016).
Lin, L. et al. Nanodrug with ROS and pH dual-sensitivity ameliorates liver fibrosis via multicellular regulation. Adv. Sci. 7, 1903138 (2020).
Zhang, D., Fan, Y., Chen, H., Trepout, S. & Li, M. H. CO2-activated reversible transition between polymersomes and micelles with AIE fluorescence. Angew. Chem. Int. Ed. 58, 10260–10265 (2019).
Suk, J. S., Xu, Q., Kim, N., Hanes, J. & Ensign, L. M. PEGylation as a strategy for improving nanoparticle-based drug and gene delivery. Adv. Drug Deliv. Rev. 99, 28–51 (2016).
Hu, J. et al. Long circulating polymeric nanoparticles for gene/drug delivery. Curr. Drug Metab. 19, 723–738 (2018).
Zhang, Z. et al. Circulatory disturbance of rat spinal cord induced by occluding ligation of the dorsal spinal vein. Acta Neuropathol. 102, 335–338 (2001).
Farrar, M. J., Rubin, J. D., Diago, D. M. & Schaffer, C. B. Characterization of blood flow in the mouse dorsal spinal venous system before and after dorsal spinal vein occlusion. J. Cereb. Blood Flow. Metab. 35, 667–675 (2015).
Bartanusz, V., Jezova, D., Alajajian, B. & Digicaylioglu, M. The blood–spinal cord barrier: morphology and clinical implications. Ann. Neurol. 70, 194–206 (2011).
Jin, L. Y. et al. Blood–spinal cord barrier in spinal cord injury: a review. J. Neurotrauma 38, 1203–1224 (2021).
Zrzavy, T. et al. Acute and non-resolving inflammation associate with oxidative injury after human spinal cord injury. Brain 144, 144–161 (2021).
Cooney, S. J., Zhao, Y. & Byrnes, K. R. Characterization of the expression and inflammatory activity of NADPH oxidase after spinal cord injury. Free Radic. Res. 48, 929–939 (2014).
Bakh, N. A. et al. Glucose-responsive insulin by molecular and physical design. Nat. Chem. 9, 937–943 (2017).
Chou, D. H. et al. Glucose-responsive insulin activity by covalent modification with aliphatic phenylboronic acid conjugates. Proc. Natl Acad. Sci. USA 112, 2401–2406 (2015).
Ahuja, C. S. et al. Traumatic spinal cord injury. Nat. Rev. Dis. Prim. 3, 17018 (2017).
Li, X. et al. The effect of a nanofiber-hydrogel composite on neural tissue repair and regeneration in the contused spinal cord. Biomaterials 245, 119978 (2020).
Schucht, P., Raineteau, O., Schwab, M. E. & Fouad, K. Anatomical correlates of locomotor recovery following dorsal and ventral lesions of the rat spinal cord. Exp. Neurol. 176, 143–153 (2002).
Qiao, Y. et al. Spinal dopaminergic mechanisms regulating the micturition reflex in male rats with complete spinal cord injury. J. Neurotrauma 38, 803–817 (2021).
Shi, Y. et al. Effective repair of traumatically injured spinal cord by nanoscale block copolymer micelles. Nat. Nanotechnol. 5, 80–87 (2010).
Ye, J. et al. Rationally designed, self-assembling, multifunctional hydrogel depot repairs severe spinal cord injury. Adv. Health. Mater. 10, e2100242 (2021).
Watson, C. et al. in The Spinal Cord Ch 15 (Academic Press, 2008).
Hong, L. T. A. et al. An injectable hydrogel enhances tissue repair after spinal cord injury by promoting extracellular matrix remodeling. Nat. Commun. 8, 533 (2017).
Basso, D. M., Beattie, M. S. & Bresnahan, J. C. Graded histological and locomotor outcomes after spinal cord contusion using the NYU weight-drop device versus transection. Exp. Neurol. 139, 244–256 (1996).
Wenger, N. et al. Spatiotemporal neuromodulation therapies engaging muscle synergies improve motor control after spinal cord injury. Nat. Med. 22, 138–145 (2016).
- SEO Powered Content & PR Distribution. Get Amplified Today.
- EVM Finance. Unified Interface for Decentralized Finance. Access Here.
- Quantum Media Group. IR/PR Amplified. Access Here.
- PlatoAiStream. Web3 Data Intelligence. Knowledge Amplified. Access Here.
- Source: https://www.nature.com/articles/s41565-023-01416-0
- :is
- ][p
- 1
- 10
- 11
- 12
- 13
- 14
- 15%
- 16
- 17
- 1996
- 1999
- 20
- 2001
- 2005
- 2008
- 2011
- 2012
- 2013
- 2014
- 2015
- 2016
- 2017
- 2018
- 2019
- 2020
- 2021
- 22
- 23
- 24
- 25
- 26
- 27
- 28
- 30
- 31
- 32
- 39
- 3d
- 40
- 46
- 49
- 7
- 8
- 9
- a
- academic
- activated
- activity
- advances
- After
- AL
- Alzheimer’s
- among
- an
- and
- architecture
- ARE
- article
- AS
- Associate
- associated
- At
- atlas
- barrier
- based
- before
- between
- biology
- Biomaterials
- Block
- blood
- by
- cell
- Cells
- chen
- circulating
- click
- Clinical
- complete
- contraction
- control
- controlled
- COVALENT
- Death
- Decoding
- defined
- Defines
- delivery
- Design
- designed
- device
- differences
- Disease
- diseases
- drug
- during
- dynamic
- e
- E&T
- Early
- ed
- effect
- Effective
- effects
- engaging
- enhance
- Enhances
- Ether (ETH)
- expression
- fan
- flow
- Focus
- following
- For
- Frequency
- function
- functional
- Health
- Hong
- http
- HTTPS
- human
- implications
- improve
- improving
- in
- increased
- individual
- inflammation
- inflammatory
- input
- ITS
- Kim
- levels
- li
- LINK
- links
- Liver
- Long
- Matrix
- measured
- mechanisms
- mice
- microglia
- model
- molecular
- motion
- Motor
- nanotechnology
- Nature
- networks
- Neural
- neuronal
- Neurons
- novel
- NYU
- of
- on
- organization
- outcomes
- oxidative
- Oxygen
- physical
- platform
- plato
- Plato Data Intelligence
- PlatoData
- Polymers
- possible
- press
- Production
- promising
- promote
- promotes
- promoting
- RAT
- recovery
- regeneration
- Regulation
- Relay
- renders
- repair
- responses
- responsive
- Reveals
- review
- Role
- s
- SC
- SCI
- searching
- severe
- simplicity
- Source
- Stem
- stem cells
- strategies
- Strategy
- system
- Technology
- that
- The
- their
- therapeutics
- therapies
- therapy
- to
- transition
- treatment
- types
- using
- Versus
- via
- voluntary
- W
- with
- X
- zephyrnet
- Zhao