1Fizikai Iskola, Melbourne-i Egyetem, Parkville, VIC 3010, Ausztrália
2Matematikai és Statisztikai Iskola, Melbourne-i Egyetem, Parkville, VIC 3010, Ausztrália
Érdekesnek találja ezt a cikket, vagy szeretne megvitatni? Scite vagy hagyjon megjegyzést a SciRate-en.
Absztrakt
A hibatűrésig a kvantumszámítás hasznosságát az határozza meg, hogy a zaj hatásait mennyire lehet megfelelően megkerülni kvantumalgoritmusokkal. A hibrid kvantum-klasszikus algoritmusokat, például a variációs kvantum-sajátmegoldót (VQE) a rövid távú rendszerhez tervezték. A problémák méretének növekedésével azonban a VQE eredményeket általában összezavarja a zaj a mai hardvereken. Míg a hibacsökkentő technikák bizonyos mértékig enyhítik ezeket a problémákat, sürgető szükség van olyan algoritmikus megközelítések kidolgozására, amelyek nagyobb robusztusságú zajjal szemben. Itt feltárjuk a nemrégiben bevezetett kvantumszámított momentumok (QCM) megközelítés robusztussági tulajdonságait az alapállapot-energia problémákra, és egy analitikus példán keresztül bemutatjuk, hogyan szűri ki az alapul szolgáló energiabecslés kifejezetten az inkoherens zajt. Ez a megfigyelés motiválja a QCM-et a kvantummágnesesség modelljéhez az IBM Quantum hardveren, hogy megvizsgáljuk a zajszűrő hatást az áramköri mélység növekedésével. Azt találtuk, hogy a QCM figyelemreméltóan magas fokú hiba robusztusságot tart fenn ott, ahol a VQE teljesen meghibásodik. A kvantummágnesesség-modell legfeljebb 20 qubites, ultramély próbaállapotú, legfeljebb 500 CNOT-os áramkörök esetében a QCM továbbra is képes ésszerű energiabecsléseket kinyerni. A megfigyelést számos kísérleti eredmény támasztja alá. Ahhoz, hogy megfeleljen ezeknek az eredményeknek, a VQE-nek körülbelül 2 nagyságrenddel hardverfejlesztésre van szüksége a hibaarányt illetően.
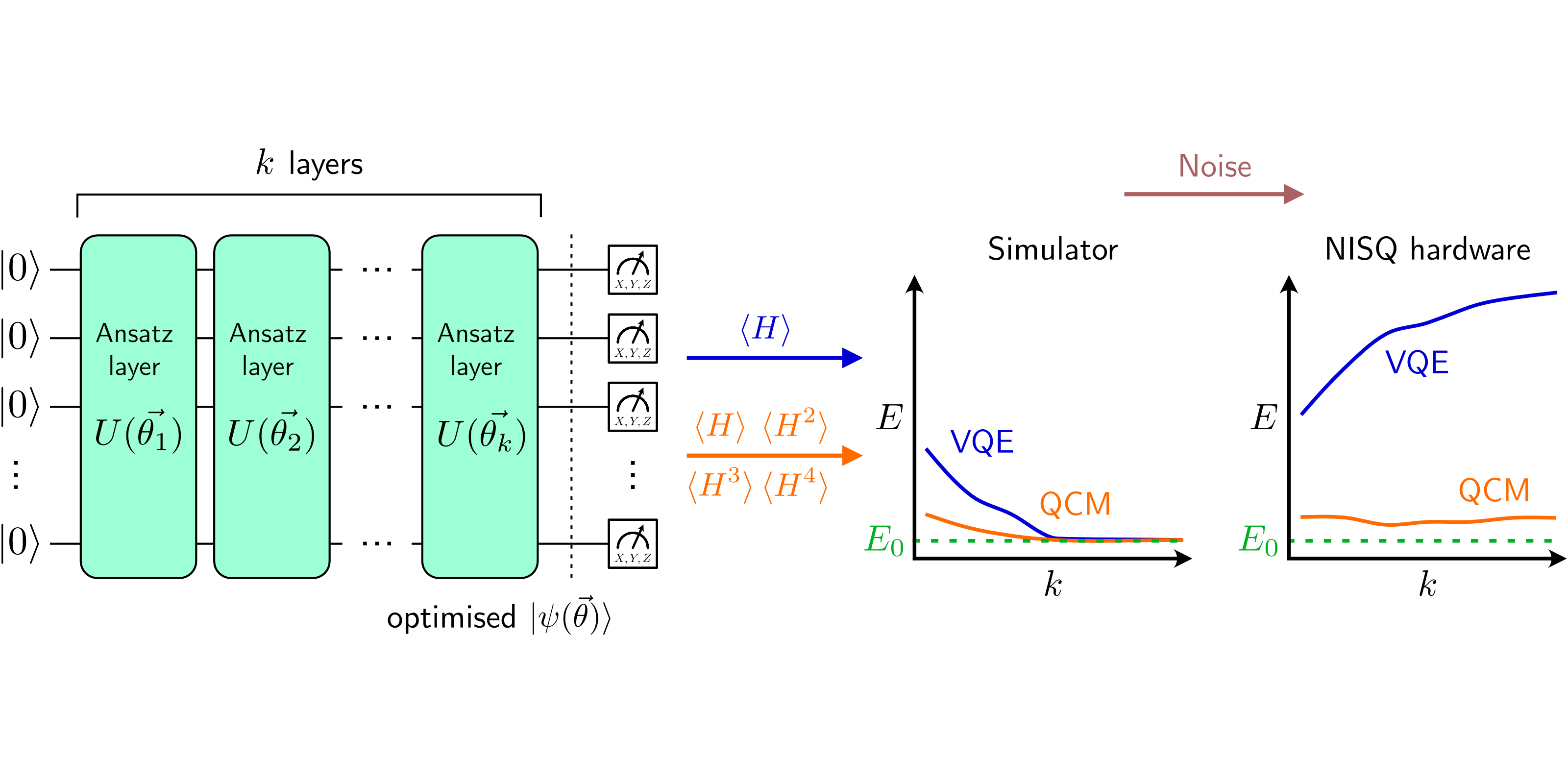
Népszerű összefoglaló
Eredményeink azt mutatják, hogy a pillanat-alapú technika figyelemre méltó szűrőhatása úgy tűnik, hogy megkerüli a zaj hatásait a mai kvantumszámítástechnika magjában, és utat mutat a hardver gyakorlati kvantumelőnyének rövid távon történő elérésére.
► BibTeX adatok
► Referenciák
[1] Sepehr Ebadi, Tout T Wang, Harry Levine, Alexander Keesling, Giulia Semeghini, Ahmed Omran, Dolev Bluvstein, Rhine Samajdar, Hannes Pichler, Wen Wei Ho, et al. “Quantum phases of matter on a 256-atom programmable quantum simulator”. Nature 595, 227–232 (2021). url: https://doi.org/10.1038/s41586-021-03582-4.
https://doi.org/10.1038/s41586-021-03582-4
[2] Xiao Mi, Pedram Roushan, Chris Quintana, Salvatore Mandra, Jeffrey Marshall, Charles Neill, Frank Arute, Kunal Arya, Juan Atalaya, Ryan Babbush, et al. “Information scrambling in quantum circuits”. Science 374, 1479–1483 (2021). url: https://doi.org/10.1126/science.abg5029.
https:///doi.org/10.1126/science.abg5029
[3] Gary J Mooney, Gregory AL White, Charles D Hill, and Lloyd CL Hollenberg. “Whole-Device Entanglement in a 65-Qubit Superconducting Quantum Computer”. Advanced Quantum Technologies 4, 2100061 (2021). url: https://doi.org/10.1002/qute.202100061.
https:///doi.org/10.1002/qute.202100061
[4] Philipp Frey and Stephan Rachel. “Realization of a discrete time crystal on 57 qubits of a quantum computer”. Science Advances 8, eabm7652 (2022). url: https://doi.org/10.1126/sciadv.abm7652.
https:///doi.org/10.1126/sciadv.abm7652
[5] Ashley Montanaro. “Quantum algorithms: an overview”. npj Quantum Information 2, 1–8 (2016). url: https://doi.org/10.1038/npjqi.2015.23.
https:///doi.org/10.1038/npjqi.2015.23
[6] Peter W Shor. “Algorithms for quantum computation: discrete logarithms and factoring”. In Proceedings 35th annual symposium on foundations of computer science. Pages 124–134. IEEE (1994). url: https://doi.org/10.1109/SFCS.1994.365700.
https:///doi.org/10.1109/SFCS.1994.365700
[7] Craig Gidney and Martin Ekerå. “How to factor 2048 bit RSA integers in 8 hours using 20 million noisy qubits”. Quantum 5, 433 (2021). url: https://doi.org/10.22331/q-2021-04-15-433.
https://doi.org/10.22331/q-2021-04-15-433
[8] Alán Aspuru-Guzik, Anthony D Dutoi, Peter J Love, and Martin Head-Gordon. “Simulated quantum computation of molecular energies”. Science 309, 1704–1707 (2005). url: https://doi.org/10.1126/science.1113479.
https:///doi.org/10.1126/science.1113479
[9] John Preskill. “Quantum computing in the NISQ era and beyond”. Quantum 2, 79 (2018). url: https://doi.org/10.22331/q-2018-08-06-79.
https://doi.org/10.22331/q-2018-08-06-79
[10] Jay Gambetta. “IBM’s roadmap for scaling quantum technology” (2020).
[11] M Morgado and S Whitlock. “Quantum simulation and computing with Rydberg-interacting qubits”. AVS Quantum Science 3, 023501 (2021). url: https://doi.org/10.1116/5.0036562.
https:///doi.org/10.1116/5.0036562
[12] Frank Arute, Kunal Arya, Ryan Babbush, Dave Bacon, Joseph C Bardin, Rami Barends, Rupak Biswas, Sergio Boixo, Fernando GSL Brandao, David A Buell, et al. “Quantum supremacy using a programmable superconducting processor”. Nature 574, 505–510 (2019). url: https://doi.org/10.1038/s41586-019-1666-5.
https://doi.org/10.1038/s41586-019-1666-5
[13] Han-Sen Zhong, Hui Wang, Yu-Hao Deng, Ming-Cheng Chen, Li-Chao Peng, Yi-Han Luo, Jian Qin, Dian Wu, Xing Ding, Yi Hu, et al. “Quantum computational advantage using photons”. Science 370, 1460–1463 (2020). url: https://doi.org/10.1126/science.abe8770.
https:///doi.org/10.1126/science.abe8770
[14] Andrew J Daley, Immanuel Bloch, Christian Kokail, Stuart Flannigan, Natalie Pearson, Matthias Troyer, and Peter Zoller. “Practical quantum advantage in quantum simulation”. Nature 607, 667–676 (2022). url: https://doi.org/10.1038/s41586-022-04940-6.
https://doi.org/10.1038/s41586-022-04940-6
[15] Iulia M Georgescu, Sahel Ashhab, and Franco Nori. “Quantum simulation”. Reviews of Modern Physics 86, 153 (2014). url: https://doi.org/10.1103/RevModPhys.86.153.
https:///doi.org/10.1103/RevModPhys.86.153
[16] Abhinav Kandala, Antonio Mezzacapo, Kristan Temme, Maika Takita, Markus Brink, Jerry M Chow, and Jay M Gambetta. “Hardware-efficient variational quantum eigensolver for small molecules and quantum magnets”. Nature 549, 242–246 (2017). url: https://doi.org/10.1038/nature23879.
https:///doi.org/10.1038/nature23879
[17] Yudong Cao, Jonathan Romero, Jonathan P Olson, Matthias Degroote, Peter D Johnson, Mária Kieferová, Ian D Kivlichan, Tim Menke, Borja Peropadre, Nicolas PD Sawaya, et al. “Quantum chemistry in the age of quantum computing”. Chemical reviews 119, 10856–10915 (2019). url: https://doi.org/10.1021/acs.chemrev.8b00803.
https:///doi.org/10.1021/acs.chemrev.8b00803
[18] Alberto Peruzzo, Jarrod McClean, Peter Shadbolt, Man-Hong Yung, Xiao-Qi Zhou, Peter J Love, Alán Aspuru-Guzik, and Jeremy L O’brien. “A variational eigenvalue solver on a photonic quantum processor”. Nature communications 5, 1–7 (2014). url: https://doi.org/10.1038/ncomms5213.
https:///doi.org/10.1038/ncomms5213
[19] Dmitry A Fedorov, Bo Peng, Niranjan Govind, and Yuri Alexeev. “VQE method: A short survey and recent developments”. Materials Theory 6, 1–21 (2022). url: https://doi.org/10.1186/s41313-021-00032-6.
https://doi.org/10.1186/s41313-021-00032-6
[20] Harper R Grimsley, Sophia E Economou, Edwin Barnes, and Nicholas J Mayhall. “An adaptive variational algorithm for exact molecular simulations on a quantum computer”. Nature communications 10, 1–9 (2019). url: https://doi.org/10.1038/s41467-019-10988-2.
https://doi.org/10.1038/s41467-019-10988-2
[21] Ho Lun Tang, VO Shkolnikov, George S Barron, Harper R Grimsley, Nicholas J Mayhall, Edwin Barnes, and Sophia E Economou. “qubit-adapt-vqe: An adaptive algorithm for constructing hardware-efficient ansätze on a quantum processor”. PRX Quantum 2, 020310 (2021). url: https://doi.org/10.1103/PRXQuantum.2.020310.
https:///doi.org/10.1103/PRXQuantum.2.020310
[22] Bryan T Gard, Linghua Zhu, George S Barron, Nicholas J Mayhall, Sophia E Economou, and Edwin Barnes. “Efficient symmetry-preserving state preparation circuits for the variational quantum eigensolver algorithm”. npj Quantum Information 6, 1–9 (2020). url: https://doi.org/10.1038/s41534-019-0240-1.
https://doi.org/10.1038/s41534-019-0240-1
[23] Kazuhiro Seki, Tomonori Shirakawa, and Seiji Yunoki. “Symmetry-adapted variational quantum eigensolver”. Physical Review A 101, 052340 (2020). url: https://doi.org/10.1103/PhysRevA.101.052340.
https:///doi.org/10.1103/PhysRevA.101.052340
[24] Gian-Luca R Anselmetti, David Wierichs, Christian Gogolin, and Robert M Parrish. “Local, expressive, quantum-number-preserving VQE ansätze for fermionic systems”. New Journal of Physics 23, 113010 (2021). url: https://doi.org/10.1088/1367-2630/ac2cb3.
https://doi.org/10.1088/1367-2630/ac2cb3
[25] Raffaele Santagati, Jianwei Wang, Antonio A Gentile, Stefano Paesani, Nathan Wiebe, Jarrod R McClean, Sam Morley-Short, Peter J Shadbolt, Damien Bonneau, Joshua W Silverstone, et al. “Witnessing eigenstates for quantum simulation of Hamiltonian spectra”. Science Advances 4, eaap9646 (2018). url: https://doi.org/10.1126/sciadv.aap9646.
https:///doi.org/10.1126/sciadv.aap9646
[26] Ikko Hamamura and Takashi Imamichi. “Efficient evaluation of quantum observables using entangled measurements”. npj Quantum Information 6, 1–8 (2020). url: https://doi.org/10.1038/s41534-020-0284-2.
https://doi.org/10.1038/s41534-020-0284-2
[27] Hsin-Yuan Huang, Richard Kueng, and John Preskill. “Efficient estimation of Pauli observables by derandomization”. Physical Review Letters 127, 030503 (2021). url: https://doi.org/10.1103/PhysRevLett.127.030503.
https:///doi.org/10.1103/PhysRevLett.127.030503
[28] Junyu Liu, Frederik Wilde, Antonio Anna Mele, Liang Jiang, and Jens Eisert. “Noise can be helpful for variational quantum algorithms” (2022). url: https://doi.org/10.48550/arXiv.2210.06723.
https:///doi.org/10.48550/arXiv.2210.06723
[29] Samson Wang, Enrico Fontana, Marco Cerezo, Kunal Sharma, Akira Sone, Lukasz Cincio, and Patrick J Coles. “Noise-induced barren plateaus in variational quantum algorithms”. Nature communications 12, 1–11 (2021). url: https://doi.org/10.1038/s41467-021-27045-6.
https://doi.org/10.1038/s41467-021-27045-6
[30] Enrico Fontana, Nathan Fitzpatrick, David Muñoz Ramo, Ross Duncan, and Ivan Rungger. “Evaluating the noise resilience of variational quantum algorithms”. Physical Review A 104, 022403 (2021). url: https://doi.org/10.1103/PhysRevA.104.022403.
https:///doi.org/10.1103/PhysRevA.104.022403
[31] Sebastian Brandhofer, Simon Devitt, and Ilia Polian. “Error Analysis of the Variational Quantum Eigensolver Algorithm”. In 2021 IEEE/ACM International Symposium on Nanoscale Architectures (NANOARCH). Pages 1–6. IEEE (2021). url: https://doi.org/10.1109/NANOARCH53687.2021.9642249.
https:///doi.org/10.1109/NANOARCH53687.2021.9642249
[32] Peter J J O’Malley, Ryan Babbush, Ian D Kivlichan, Jonathan Romero, Jarrod R McClean, Rami Barends, Julian Kelly, Pedram Roushan, Andrew Tranter, Nan Ding, et al. “Scalable quantum simulation of molecular energies”. Physical Review X 6, 031007 (2016). url: https://doi.org/10.1103/PhysRevX.6.031007.
https:///doi.org/10.1103/PhysRevX.6.031007
[33] Yangchao Shen, Xiang Zhang, Shuaining Zhang, Jing-Ning Zhang, Man-Hong Yung, and Kihwan Kim. “Quantum implementation of the unitary coupled cluster for simulating molecular electronic structure”. Physical Review A 95, 020501 (2017). url: https://doi.org/10.1103/PhysRevA.95.020501.
https:///doi.org/10.1103/PhysRevA.95.020501
[34] Frank Arute, Kunal Arya, Ryan Babbush, Dave Bacon, Joseph C Bardin, Rami Barends, Sergio Boixo, Michael Broughton, Bob B Buckley, et al. “Hartree-Fock on a superconducting qubit quantum computer”. Science 369, 1084–1089 (2020). url: https://doi.org/10.1126/science.abb9811.
https:///doi.org/10.1126/science.abb9811
[35] Seunghoon Lee, Joonho Lee, Huanchen Zhai, Yu Tong, Alexander M Dalzell, Ashutosh Kumar, Phillip Helms, Johnnie Gray, Zhi-Hao Cui, Wenyuan Liu, et al. “Is there evidence for exponential quantum advantage in quantum chemistry?” (2022). url: https://doi.org/10.48550/arXiv.2208.02199.
https:///doi.org/10.48550/arXiv.2208.02199
[36] Harish J Vallury, Michael A Jones, Charles D Hill, and Lloyd C L Hollenberg. “Quantum computed moments correction to variational estimates”. Quantum 4, 373 (2020). url: https://doi.org/10.22331/q-2020-12-15-373.
https://doi.org/10.22331/q-2020-12-15-373
[37] Lloyd C L Hollenberg. “Plaquette expansion in lattice Hamiltonian models”. Physical Review D 47, 1640 (1993). url: https://doi.org/10.1103/PhysRevD.47.1640.
https:///doi.org/10.1103/PhysRevD.47.1640
[38] Lloyd C L Hollenberg and NS Witte. “General nonperturbative estimate of the energy density of lattice Hamiltonians”. Physical Review D 50, 3382 (1994). url: https://doi.org/10.1103/PhysRevD.50.3382.
https:///doi.org/10.1103/PhysRevD.50.3382
[39] Lloyd C L Hollenberg and NS Witte. “Analytic solution for the ground-state energy of the extensive many-body problem”. Physical Review B 54, 16309 (1996). url: https://doi.org/10.1103/PhysRevB.54.16309.
https:///doi.org/10.1103/PhysRevB.54.16309
[40] Michael A Jones, Harish J Vallury, Charles D Hill, and Lloyd C L Hollenberg. “Chemistry beyond the Hartree–Fock energy via quantum computed moments”. Scientific Reports 12, 1–9 (2022). url: https://doi.org/10.1038/s41598-022-12324-z.
https:///doi.org/10.1038/s41598-022-12324-z
[41] Edward Farhi, Jeffrey Goldstone és Sam Gutmann. „A kvantumközelítő optimalizálási algoritmus” (2014). url: https:///doi.org/10.48550/arXiv.1411.4028.
https:///doi.org/10.48550/arXiv.1411.4028
[42] Aochen Duan. “Matrix product states in quantum information processing”. Master’s thesis. School of Physics, The University of Melbourne. (2015).
[43] Michael A. Jones. “Moments-based corrections to variational quantum computation”. Master’s thesis. School of Physics, The University of Melbourne. (2019).
[44] Karol Kowalski and Bo Peng. “Quantum simulations employing connected moments expansions”. The Journal of Chemical Physics 153, 201102 (2020). url: https://doi.org/10.1063/5.0030688.
https:///doi.org/10.1063/5.0030688
[45] Kazuhiro Seki and Seiji Yunoki. “Quantum power method by a superposition of time-evolved states”. PRX Quantum 2, 010333 (2021). url: https://doi.org/10.1103/PRXQuantum.2.010333.
https:///doi.org/10.1103/PRXQuantum.2.010333
[46] Philippe Suchsland, Francesco Tacchino, Mark H Fischer, Titus Neupert, Panagiotis Kl Barkoutsos, and Ivano Tavernelli. “Algorithmic error mitigation scheme for current quantum processors”. Quantum 5, 492 (2021). url: https://doi.org/10.22331/q-2021-07-01-492.
https://doi.org/10.22331/q-2021-07-01-492
[47] Joseph C Aulicino, Trevor Keen, and Bo Peng. “State preparation and evolution in quantum computing: A perspective from Hamiltonian moments”. International Journal of Quantum Chemistry 122, e26853 (2022). url: https://doi.org/10.1002/qua.26853.
https:///doi.org/10.1002/qua.26853
[48] Lloyd C L Hollenberg, David C Bardos, and NS Witte. “Lanczos cluster expansion for non-extensive systems”. Zeitschrift für Physik D Atoms, Molecules and Clusters 38, 249–252 (1996). url: https://doi.org/10.1007/s004600050089.
https:///doi.org/10.1007/s004600050089
[49] David Horn and Marvin Weinstein. “The t expansion: A nonperturbative analytic tool for Hamiltonian systems”. Physical Review D 30, 1256 (1984). url: https://doi.org/10.1103/PhysRevD.30.1256.
https:///doi.org/10.1103/PhysRevD.30.1256
[50] Calvin Stubbins. “Methods of extrapolating the t-expansion series”. Physical Review D 38, 1942 (1988). url: https://doi.org/10.1103/PhysRevD.38.1942.
https:///doi.org/10.1103/PhysRevD.38.1942
[51] J Cioslowski. “Connected moments expansion: a new tool for quantum many-body theory”. Physical review letters 58, 83 (1987). url: https://doi.org/10.1103/PhysRevLett.58.83.
https:///doi.org/10.1103/PhysRevLett.58.83
[52] Alexander M Dalzell, Nicholas Hunter-Jones, and Fernando GSL Brandão. “Random quantum circuits transform local noise into global white noise” (2021). url: https://doi.org/10.48550/arXiv.2111.14907.
https:///doi.org/10.48550/arXiv.2111.14907
[53] NS Witte and Lloyd C L Hollenberg. “Accurate calculation of ground-state energies in an analytic Lanczos expansion”. Journal of Physics: Condensed Matter 9, 2031 (1997). url: https://doi.org/10.1088/0953-8984/9/9/016.
https://doi.org/10.1088/0953-8984/9/9/016
[54] Qiskit közreműködők. „Qiskit: Nyílt forráskódú keretrendszer a kvantumszámításhoz” (2023).
[55] Suguru Endo, Simon C Benjamin, and Ying Li. “Practical quantum error mitigation for near-future applications”. Physical Review X 8, 031027 (2018). url: https://doi.org/10.1103/PhysRevX.8.031027.
https:///doi.org/10.1103/PhysRevX.8.031027
[56] Tudor Giurgica-Tiron, Yousef Hindy, Ryan LaRose, Andrea Mari, and William J Zeng. “Digital zero noise extrapolation for quantum error mitigation”. In 2020 IEEE International Conference on Quantum Computing and Engineering (QCE). Pages 306–316. IEEE (2020). url: https://doi.org/10.1109/QCE49297.2020.00045.
https:///doi.org/10.1109/QCE49297.2020.00045
[57] Kristan Temme, Sergey Bravyi, and Jay M Gambetta. “Error mitigation for short-depth quantum circuits”. Physical review letters 119, 180509 (2017). url: https://doi.org/10.1103/PhysRevLett.119.180509.
https:///doi.org/10.1103/PhysRevLett.119.180509
[58] Sergey Bravyi, Sarah Sheldon, Abhinav Kandala, David C Mckay, and Jay M Gambetta. “Mitigating measurement errors in multiqubit experiments”. Physical Review A 103, 042605 (2021). url: https://doi.org/10.1103/PhysRevA.103.042605.
https:///doi.org/10.1103/PhysRevA.103.042605
[59] Hendrik Weimer, Augustine Kshetrimayum, and Román Orús. “Simulation methods for open quantum many-body systems”. Reviews of Modern Physics 93, 015008 (2021). url: https://doi.org/10.1103/RevModPhys.93.015008.
https:///doi.org/10.1103/RevModPhys.93.015008
[60] Pranav Gokhale, Olivia Angiuli, Yongshan Ding, Kaiwen Gui, Teague Tomesh, Martin Suchara, Margaret Martonosi, and Frederic T Chong. “$ O (N^{3}) $ Measurement Cost for Variational Quantum Eigensolver on Molecular Hamiltonians”. IEEE Transactions on Quantum Engineering 1, 1–24 (2020). url: https://doi.org/10.1109/TQE.2020.3035814.
https:///doi.org/10.1109/TQE.2020.3035814
[61] Lloyd C L Hollenberg and Michael J Tomlinson. “Staggered magnetisation in the Heisenberg antiferromagnet”. Australian journal of physics 47, 137–144 (1994). url: https://doi.org/10.1071/PH940137.
https:///doi.org/10.1071/PH940137
Idézi
[1] Floyd M. Creevey, Charles D. Hill, and Lloyd C. L. Hollenberg, “GASP: a genetic algorithm for state preparation on quantum computers”, Scientific Reports, 13, 11956 (2023).
A fenti idézetek innen származnak SAO/NASA HIRDETÉSEK (utolsó sikeres frissítés: 2023-09-11 15:35:44). Előfordulhat, hogy a lista hiányos, mivel nem minden kiadó ad megfelelő és teljes hivatkozási adatokat.
Nem sikerült lekérni Az adatok által hivatkozott kereszthivatkozás utolsó próbálkozáskor 2023-09-11 15:35:43: Nem sikerült lekérni a 10.22331/q-2023-09-11-1109 hivatkozás által hivatkozott adatokat a Crossref-től. Ez normális, ha a DOI-t nemrég regisztrálták.
Ez a tanulmány a Quantumban jelent meg Creative Commons Nevezd meg 4.0 International (CC BY 4.0) engedély. A szerzői jog az eredeti szerzői jog tulajdonosainál marad, például a szerzőknél vagy intézményeiknél.
- SEO által támogatott tartalom és PR terjesztés. Erősödjön még ma.
- PlatoData.Network Vertical Generative Ai. Erősítse meg magát. Hozzáférés itt.
- PlatoAiStream. Web3 Intelligence. Felerősített tudás. Hozzáférés itt.
- PlatoESG. Autóipar / elektromos járművek, Carbon, CleanTech, Energia, Környezet, Nap, Hulladékgazdálkodás. Hozzáférés itt.
- PlatoHealth. Biotechnológiai és klinikai vizsgálatok intelligencia. Hozzáférés itt.
- ChartPrime. Emelje fel kereskedési játékát a ChartPrime segítségével. Hozzáférés itt.
- BlockOffsets. A környezetvédelmi ellentételezési tulajdon korszerűsítése. Hozzáférés itt.
- Forrás: https://quantum-journal.org/papers/q-2023-09-11-1109/
- :is
- :nem
- :ahol
- ][p
- $ UP
- 1
- 10
- 11
- 12
- 13
- 14
- 15%
- 16
- 17
- 19
- 1994
- 1996
- 20
- 2005
- 2014
- 2015
- 2016
- 2017
- 2018
- 2019
- 2020
- 2021
- 2022
- 2023
- 2031
- 22
- 23
- 24
- 25
- 26
- 27
- 28
- 29
- 30
- 31
- 32
- 33
- 36
- 39
- 40
- 41
- 46
- 49
- 50
- 500
- 51
- 52
- 53
- 54
- 60
- 7
- 8
- 9
- a
- Képes
- felett
- KIVONAT
- hozzáférés
- elérése
- adaptív
- További
- megfelelő
- fejlett
- előlegek
- Előny
- hovatartozás
- kor
- Ahmed
- AL
- Alexander
- algoritmus
- algoritmikus
- algoritmusok
- Minden termék
- enyhít
- an
- elemzés
- elemzés
- Analitikus
- és a
- Andrew
- Anna
- évi
- Anthony
- alkalmazások
- megközelítés
- megközelít
- hozzávetőleges
- VANNAK
- AS
- At
- ausztrál
- szerző
- szerzők
- b
- meddő
- BE
- óta
- Benjámin
- Túl
- Bit
- Bo
- gabona
- szünet
- perem
- Bryan
- de
- by
- számítás
- Calvin
- TUD
- kihívás
- Károly
- kémiai
- kémia
- chen
- chong
- ebéd
- chris
- Fürt
- megjegyzés
- Közös
- köznép
- távközlés
- teljes
- teljesen
- számítás
- számítógép
- Computer Science
- számítógépek
- számítástechnika
- Sűrített anyag
- Konferencia
- összefüggő
- építése
- közreműködők
- hagyományos
- copyright
- Mag
- kijavítására
- Hiba
- Költség
- tudott
- összekapcsolt
- Craig
- Kristály
- Jelenlegi
- dátum
- Dave
- David
- nap
- mély
- Fok
- Azt
- sűrűség
- mélység
- tervezett
- Határozzuk meg
- eltökélt
- Fejleszt
- fejlett
- fejlesztések
- eszköz
- megvitatni
- Zavar
- Duncan
- alatt
- e
- E&T
- Edward
- Edwin
- hatás
- hatások
- bármelyik
- Elektronikus
- energia
- energia sűrűség
- Mérnöki
- elég
- Ez volt
- hiba
- hibák
- becslés
- becslések
- Eter (ETH)
- értékelés
- Még
- bizonyíték
- evolúció
- megvizsgálni
- példa
- létezik
- terjeszkedés
- kísérleti
- kísérletek
- feltárása
- exponenciális
- kifejező
- kiterjedt
- mérték
- kivonat
- tényező
- nem sikerül
- mező
- szűrő
- Szűrők
- Találjon
- Fitzpatrick
- Floyd
- A
- Alapok
- Keretrendszer
- őszinte
- ból ből
- Gary
- Gates
- általános
- általában
- György
- Globális
- szürke
- legnagyobb
- Földi
- hardver
- Harvard
- Legyen
- hasznos
- itt
- Magas
- <p></p>
- tartók
- NYITVATARTÁS
- Hogyan
- azonban
- HTTPS
- huang
- hibrid
- hibrid kvantum-klasszikus
- IBM
- ibm quantum
- IEEE
- if
- végre
- végrehajtás
- javulás
- in
- Növeli
- növekvő
- információ
- eredendően
- intézmények
- érdekes
- Nemzetközi
- bele
- Bevezetett
- vonja
- kérdések
- ivan
- JavaScript
- jeffrey
- János
- Johnnie
- Johnson
- Jonatán
- jones
- joshua
- folyóirat
- juan
- Lelkes
- Kim
- Kumar
- keresztnév
- vezet
- Szabadság
- Lee
- szintek
- li
- Engedély
- Lista
- helyi
- szerelem
- Mágnesesség
- Mágnesek
- fenntartja
- Marco
- jel
- Márton
- mester
- Mérkőzés
- párosított
- anyagok
- matematika
- Anyag
- Matthias
- max-width
- Lehet..
- mcclean
- mérés
- mérések
- mérő
- Melbourne
- módszer
- mód
- Michael
- millió
- enyhítés
- modell
- modellek
- modern
- molekuláris
- Pillanatok
- Hónap
- motivált
- Természet
- Szükség
- Új
- miklós
- Nicolas
- Zaj
- normális
- of
- on
- ONE
- nyitva
- nyílt forráskódú
- optimalizálás
- or
- rendelés
- eredeti
- mi
- ki
- áttekintés
- oldalak
- Papír
- patrick
- Pearson
- perspektíva
- kimerül
- Az anyag fázisai
- Philippe
- Fotonok
- fizikai
- Fizika
- Plató
- Platón adatintelligencia
- PlatoData
- játék
- pont
- potenciálisan
- hatalom
- erős
- Gyakorlati
- előkészítés
- előkészített
- előkészítése
- be
- sürgős
- Probléma
- problémák
- Eljárás
- feldolgozás
- Processzor
- processzorok
- Termékek
- ingatlanait
- ad
- közzétett
- kiadó
- kiadók
- qiskit
- Kvantum
- kvantumelőny
- kvantum algoritmusok
- Kvantum számítógép
- kvantum számítógépek
- kvantumszámítás
- kvantuminformáció
- kvantumtechnika
- qubit
- qubit
- kérdés
- gyorsan
- R
- RÖMI
- Az árak
- igazi
- való Világ
- ésszerű
- új
- nemrég
- csökkentés
- referenciák
- rezsim
- nyilvántartott
- maradványok
- figyelemre méltó
- Jelentések
- szükség
- rugalmasság
- forrás
- eredményez
- Eredmények
- Kritika
- Vélemények
- Richard
- ütemterv
- ROBERT
- erős
- robusztusság
- rsa
- Ryan
- s
- Sam
- Skála
- skálázás
- rendszer
- Iskola
- Tudomány
- tudományos
- Series of
- készlet
- Sharma
- rövid
- rövid időszak
- előadás
- Silverstone
- Simon
- tettetés
- szimulátor
- kicsi
- megoldások
- néhány
- sophia
- Állami
- Államok
- statisztika
- Még mindig
- stratégiák
- struktúra
- sikeresen
- ilyen
- megfelelő
- ráhelyezés
- Felmérés
- Szimpózium
- Systems
- T
- csap
- technikák
- Technologies
- Technológia
- hogy
- A
- azok
- elméleti
- elmélet
- Ott.
- Ezek
- tézis
- ezt
- Keresztül
- Tim
- idő
- Cím
- nak nek
- tolerancia
- szerszám
- Végösszeg
- Tranzakciók
- Átalakítás
- Trevor
- próba
- kettő
- jellemzően
- Végül
- alatt
- mögöttes
- egyetemi
- frissítve
- felfelé
- URL
- segítségével
- hasznosság
- keresztül
- kötet
- W
- akar
- volt
- Út..
- we
- súly
- ami
- míg
- fehér
- lesz
- william
- val vel
- Munka
- világ
- lenne
- wu
- X
- xiao
- év
- IGEN
- zephyrnet
- nulla
- Zhong