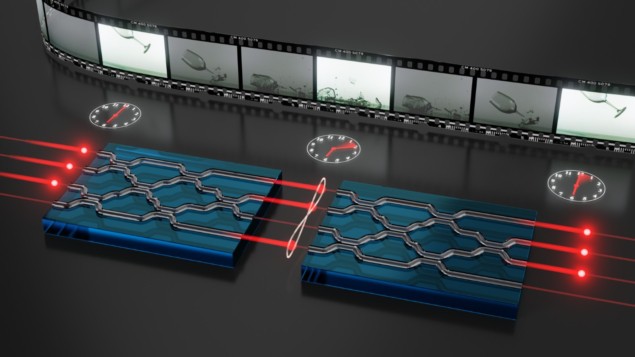
Physicists in the Netherlands and Germany have shown that the theories of thermodynamics and quantum mechanics are both valid ways of describing the behaviour of photons in a quantum processor. The results, obtained by researchers at the University of Twente and the Freie Universität Berlin, open the door to a deeper understanding of how to reconcile these two great theories.
Thermodynamics and quantum mechanics are cornerstones of modern physics, but in one specific, important way, they don’t get along well. The point of contention revolves around the second law of thermodynamics, which states that a closed system will move towards maximum entropy (a measure of the system’s disorder, or chaos) in an irreversible way. The theory of quantum mechanics, in contrast, allows previous states of particles to be calculated back, meaning that the flow of information and time are both reversible.
In recent years, there have been several attempts to explore this conflict using entangled quantum systems such as ultracold atoms or superconducting quantum bits (qubits). By observing what happens when these systems thermalize and equilibrate, it should be possible to measure their entropy and quantum states at the same time, and thus resolve the paradox.
The problem is that quantum systems are very sensitive to interactions with their environment. This makes it hard to create a system that is truly closed. They are also prone to losing their quantum nature, a process known as decoherence, which makes time reversal difficult to implement.
Photonics to the rescue
To get around these challenges, the team chose to study thermalization and equilibration in systems of entangled photons. Photons have several advantages over quantum systems composed of (for example) atoms. Their intrinsically quantum nature means they do not suffer from decoherence. They can be studied at room temperature, in contrast to the ultralow temperatures necessary for atoms, and are easy to manipulate with interference. Most importantly, they allow for time reversibility: any mixing of the photons can be reversed by performing the inverse operation, meaning that entangled photons can, in effect, be “disentangled”.
In the experiment, the researchers begin by injecting single photons into waveguide channels on a chip. These photons interfere where the photonic channels on the chip meet and cross. This interference, which the team controlled with thermo-optic Mach-Zehnder interferometers, creates a superposition of photons in the waveguides and allows entanglement to build up. The photons are then detected with single-photon detectors.
Simultaneously true
To determine the system’s local and total increases in entropy, the researchers performed a series of protocols. Time reversibility, for example, was implemented by disentangling the photons, which was possible due to the full control the processor gives over the experiment.
Once these protocols were complete, measurements in the experiment’s individual output channels showed that photon numbers could no longer be precisely defined. This is because the photons were in an entangled state together and no longer individually localized in a single channel as they were at the input. However, the photon statistics the researchers measured in each channel did show that entropy increased locally in all the channels, consistent with the second law of thermodynamics. At the same time, the entanglement that built up between photons is not visible in the individual channels: only when considering the entire system does it become clear that the overall quantum state is in a pure form, consistent with quantum mechanics.
As a final check, the physicists performed operations to return the processor to its original state (time reversal). The success of these operations proved that the processes of thermalization and equilibration were due to entanglement between the quantum particles, rather than interactions with the environment. Hence, the experiment showed that thermodynamics and quantum mechanics can both be true at the same time.
High-quality data
According to Pepijn Pinkse, a quantum optics expert at the University of Twente, the team’s biggest challenge was to get enough high quality data to perform the measurements. Low losses in the photonic processor helped, he says, and more photons and larger processors should enable them to simulate more systems. The weakest element in the chain, he adds, seems to be the photon source: “We have at least 12 input channels, but only three photons at the same time to experiment with, so there’s room for improvement there,” he tells Physics World.
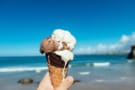
The unsung theory: why thermodynamics is as important as quantum mechanics and general relativity
Nicole Yunger Halpern, an expert in quantum thermodynamics at the US National Institute of Standards and Technology (NIST) who was not involved in the research, says the experiment is important because it extends to photons previous work that involved ultracold atoms, trapped ions and superconducting qubits. This change of platform, she says, enabled the experimentalists to undo the process that led the system to equilibrate internally, making it possible to conclude that the system had retained its quantum nature while equilibrating. Doing this requires an “excellent amount of control”, she notes, adding that the challenge of achieving this control has caused groups using other platforms significant anxiety over the past several years.
The research is published in Nature Communications.
- SEO Powered Content & PR Distribution. Get Amplified Today.
- PlatoData.Network Vertical Generative Ai. Empower Yourself. Access Here.
- PlatoAiStream. Web3 Intelligence. Knowledge Amplified. Access Here.
- PlatoESG. Automotive / EVs, Carbon, CleanTech, Energy, Environment, Solar, Waste Management. Access Here.
- BlockOffsets. Modernizing Environmental Offset Ownership. Access Here.
- Source: https://physicsworld.com/a/quantum-mechanics-and-thermodynamics-can-both-be-true-say-physicists/
- :has
- :is
- :not
- :where
- $UP
- 12
- 90
- a
- achieving
- adding
- Adds
- advantages
- All
- allow
- allows
- along
- also
- amount
- an
- and
- Anxiety
- any
- ARE
- around
- AS
- At
- Attempts
- back
- BE
- Beach
- because
- become
- been
- begin
- berlin
- between
- Biggest
- both
- Bottom
- build
- built
- but
- by
- calculated
- CAN
- caused
- chain
- challenge
- challenges
- change
- Channel
- channels
- Chaos
- check
- chip
- chose
- clear
- Clocks
- closed
- complete
- composed
- conclude
- conflict
- considering
- consistent
- contrast
- control
- controlled
- cornerstones
- could
- Cream
- create
- creates
- Cross
- data
- deeper
- defined
- detected
- Determine
- DID
- difficult
- disorder
- do
- does
- doing
- Dont
- Door
- due
- each
- easy
- effect
- element
- enable
- enabled
- enough
- Entire
- Environment
- example
- experiment
- expert
- explore
- Explored
- extends
- final
- flow
- For
- form
- from
- full
- General
- Germany
- get
- gives
- glass
- great
- Group’s
- had
- happens
- Hard
- Have
- he
- helped
- hence
- High
- How
- How To
- However
- HTTPS
- ICE
- ice cream
- image
- images
- implement
- implemented
- important
- improvement
- in
- increased
- Increases
- increasing
- individual
- Individually
- information
- input
- Institute
- interactions
- interfere
- internally
- into
- intrinsically
- involved
- issue
- IT
- ITS
- jpg
- known
- larger
- Law
- least
- Led
- light
- local
- locally
- longer
- losing
- losses
- Low
- MAKES
- Making
- max-width
- maximum
- meaning
- means
- measure
- measured
- measurements
- mechanics
- Meet
- Middle
- Mixing
- Modern
- more
- most
- move
- movie
- National
- Nature
- necessary
- Netherlands
- nist
- no
- Notes
- numbers
- obtained
- of
- on
- ONE
- only
- open
- operation
- Operations
- optics
- or
- original
- Other
- output
- over
- overall
- Paradox
- past
- perform
- performed
- performing
- Photons
- Physics
- Physics World
- platform
- Platforms
- plato
- Plato Data Intelligence
- PlatoData
- Point
- possible
- precisely
- previous
- Problem
- process
- processes
- Processor
- processors
- protocols
- proved
- published
- quality
- quality data
- Quantum
- Quantum Mechanics
- Quantum optics
- quantum particles
- quantum systems
- qubits
- rather
- recent
- relationship
- represent
- represented
- representing
- requires
- research
- researchers
- Results
- return
- Reversal
- revolves
- Room
- s
- same
- say
- says
- Second
- seems
- sensitive
- Series
- several
- she
- should
- show
- showed
- shown
- significant
- single
- So
- Source
- specific
- standards
- State
- States
- statistics
- studied
- Study
- success
- such
- superposition
- system
- Systems
- team
- Technology
- tells
- than
- that
- The
- the Netherlands
- their
- Them
- then
- theory
- There.
- These
- they
- this
- three
- Through
- thumbnail
- Thus
- time
- to
- together
- top
- Total
- towards
- true
- truly
- two
- understanding
- university
- us
- using
- very
- visible
- W
- was
- Way..
- ways
- WELL
- were
- What
- when
- which
- while
- WHO
- why
- will
- with
- Work
- world
- years
- zephyrnet