Rare-earth elements are vital for the magnets found in electric cars, wind turbines and other parts of the “green economy”. But with uncertainties over the supply of these materials, James McKenzie reports on the importance of magnets that avoid rare earths entirely
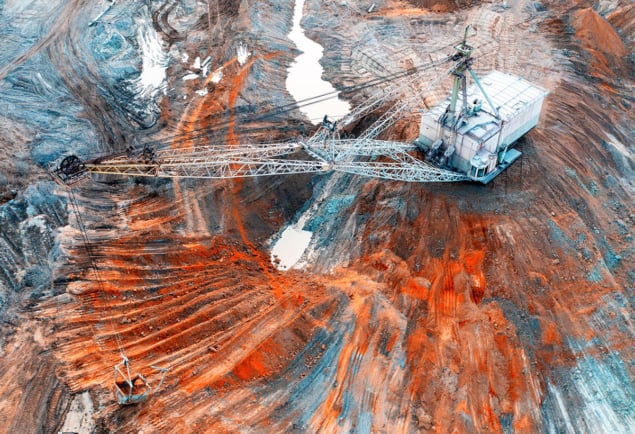
Magnets might not be something on most people’s minds, but they are essential for the burgeoning “green economy”, lying at the heart of motors for electric vehicles and generators in wind turbines. Demand is rising particularly for the powerful, permanent magnets made from alloys of “rare-earth” elements. But with uncertainty over the continued supply of rare earths, the search is on for alternative magnets that perform as well but are completely made from other elements.
In case you’ve forgotten your chemistry, rare-earth elements consist of the lanthanides, which are in the long horizontal part of the periodic table, along with the non-lanthanides yttrium and scandium in group 3. When it comes to magnets, of most interest are neodymium, samarium and cerium as well as the “heavy” rare earths – dysprosium, terbium and ytterbium. The strongest and most used permanent magnets, however, are alloys of neodymium, iron and cobalt (NdFeB) and samarium cobalt (SmCo).
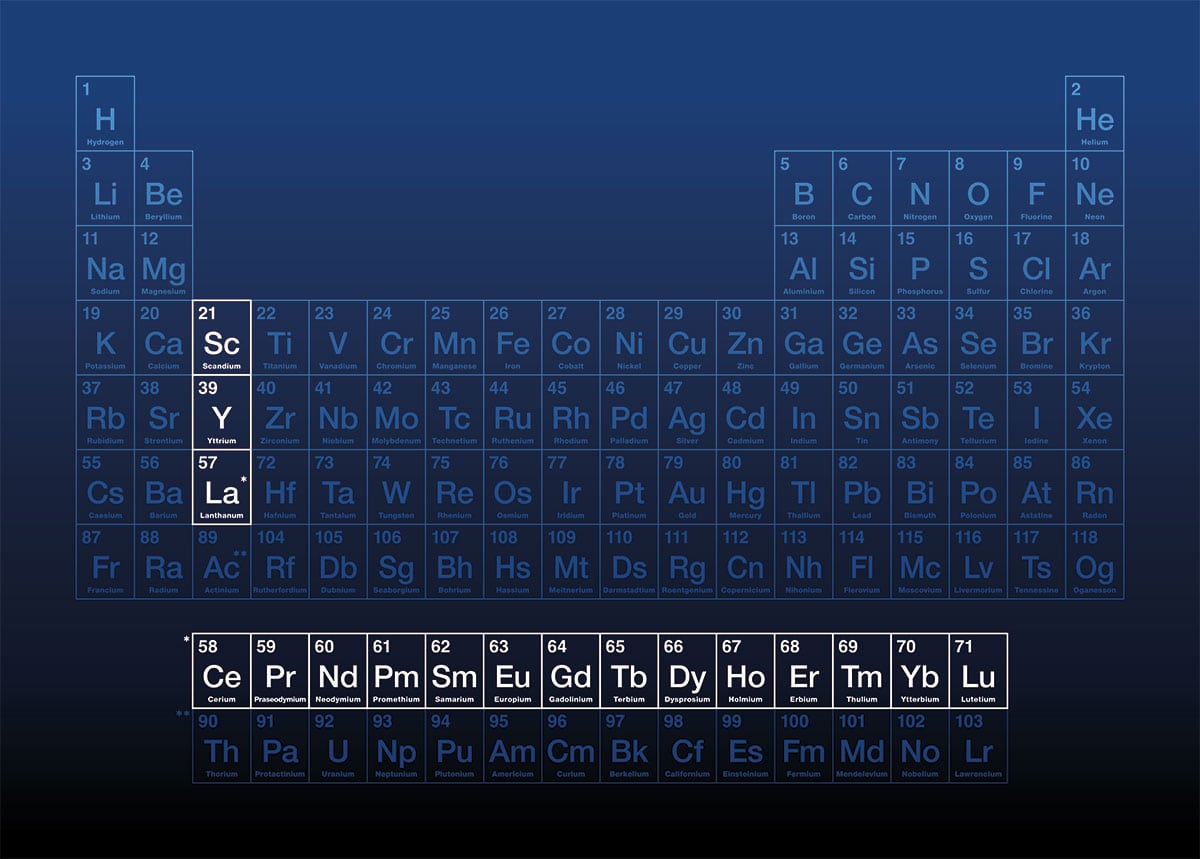
Rare earths are relatively abundant, with over 160 minerals known to contain them. Trouble is, they occur in such miniscule concentrations that only four minerals are mined for their rare earths, the others being too expensive to recover. Bastnäsite is the principal source of rare earths – accounting for 94% of supply – and is the world’s main source of neodymium magnets. Laterite clays, meanwhile, are the main commercial source of heavy rare earths.
Separating and refining rare earths is also difficult because they are chemically very similar. It’s environmentally costly too, although that hasn’t stopped demand for permanent magnets from surging. A report from Magnetics & Materials suggests that by 2030 the world will need 55,000 more tonnes of neodymium magnets than are likely to be available. Indeed, the overall magnet market, which was worth $29.3 in 2021, is set to grow by almost 6% a year to 2030, according a recent analysis from Grand View Research.
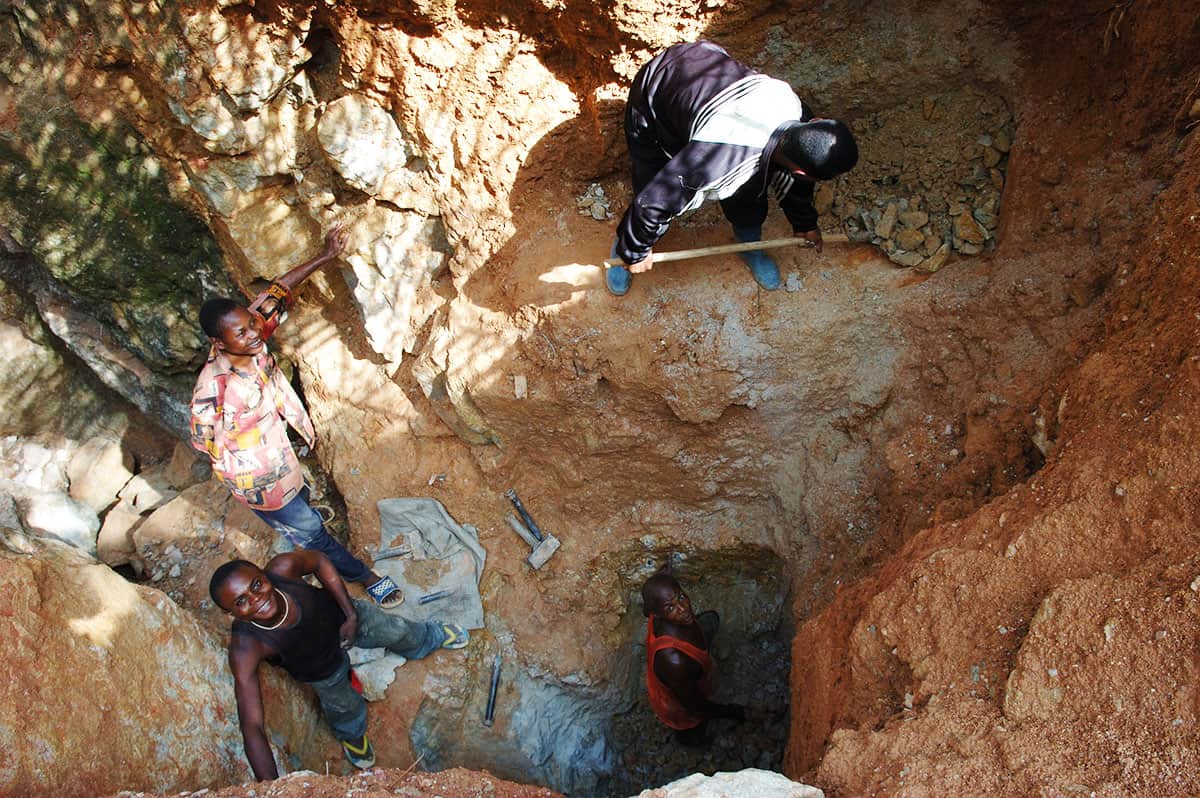
Demand for SmCo magnets is rising too, despite ethical and environmental concerns over how cobalt is mined in the Democratic Republic of Congo, which has the world’s largest reserves of the element (most samarium comes from China). A new report from Amnesty International, for example, says that the expansion of cobalt and copper mining in the country has led to communities being forcibly evicted along with human rights abuse “including sexual assault, arson and beatings”.
Many of the challenges of finding and making non-rare-earth magnets were discussed at the REPM23 conference held at the University of Birmingham in the UK in September. Known officially as the 27th International Workshop on Rare Earth and Future Permanent Magnets and their Applications, the meeting featured the great and the good from academia and industry from around the world. As I discovered, there is a huge amount of exciting and important research and technology going on in the field.
Seeking performance
From a physics point of view, the beauty of permanent magnets is that they store lots of energy, which allows small and highly efficient devices to be made from them. In general, the higher the magnet performance, the higher the motor efficiency. So even though high-performance magnets are expensive, it’s worth the extra cost because less money needs to be spent on other parts of the system in which they’re used. A more efficient motor, for example, means that the expensive battery in an electric vehicle doesn’t need to be quite so large.

The overall performance of a permanent magnet is therefore vital, with the key figure of merit being the amount of energy you can store in the material. Known as the “maximum energy product”, or BHmax, it is roughly 38 kJ/m3 for ferrite (BaFeO), which is the cheapest magnetic material at about $3–6 per kilogram. But for high-performance neodymium magnets, which cost about $40–80 per kilogram, the BHmax is a much larger 410 kJ/m3.
But being cheap and having a high energy product isn’t everything. Companies designing motors or generators also want a magnet that can generate a large magnetic field (i.e. with a big “remanence”). In addition, the magnet needs to have a high “coercivity”, which is essentially a measure of how much energy is needed to demagnetize it. Coercivity depends on how a magnet is made, with key factors including the size of crystals and the number and amount of additives used to toughen the material. If the coercivity is too low, the magnet will lose its energy, demagnetize and make the motor or generator useless.
Another vital factor is a magnet’s Curie temperature (Tc), above which its magnetism is lost. Neodymium magnets have a relatively low Tc of about 210 oC, which is fine for most applications. But samarium cobalt has a Tc of up to 800 oC, making these magnets great in motor sport and other applications where high temperatures are commonplace. AlNiCo – an alloy of aluminium, nickel and cobalt – is the only mainstream material with a Tc higher than samarium cobalt (1000 oC) and a BHmax better than ferrite (at 310 kJ/m3), but its coercivity is so poor that it’s of limited use, especially now that higher coercivity magnets exist.
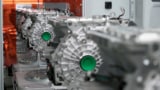
Magnets, magnets, magnets: we’ll need lots of them for a green economy
People in the magnet community have therefore long been seeking a magnet that sits in the gap between ferrite and neodymium in terms of price and performance. Everyone would love a super-cheap magnet that outperforms neodymium but these “god-like” materials don’t currently exist – in fact, some say they never will. Still, every time the price of neodymium spikes, there’s a resurgence of interest in new magnets. In fact, I was shocked to learn at the Birmingham meeting just how many potential magnet materials are in the running.
To me at least, it seems that the challenge isn’t in making new materials per se. What’s difficult is optimizing the material and the production process, which can literally take decades. As the Japanese scientist Masato Sagawa – the inventor of neodymium magnets – pointed out in the opening plenary at REPM, it has taken 40 years of heroic effort for these materials to reach their current BHmax, which is about 90% of its theoretical maximum value, and to achieve high coercivity and high performance.
A matter of choice
One cheaper alternative to neodymium is cerium, which is dug up and refined at the same time. It could partially replace the neodymium in NdFeB magnets, reducing costs but with a drop in performance. However, there are some other promising types of magnet that are much less polluting and use no rare earths altogether. If we can get these going, we’d really be turning base metals into modern-day “green gold”.
One of the most promising and well backed seems to be iron nitride (FeN). Based on just two materials – iron and nitrogen – that are cheap and in plentiful supply, it has a BHmax of 1150 kJ/m3 and a Tc of 540 oC. Firms like Niron in California are already investing substantially in the area, employing a large and growing number of materials scientists to fine-tune its properties and production.
Another contender is manganese aluminium carbon (MnAlC), which was originally commercialized in the 1980s before being abandoned as neodymium magnets came to the fore. Materials physicists at Sheffield University, led by Elizabeth Davis-Fowell, have even recently shown that MnAlGa, which replaces the carbon in MnAlC with gallium, could be better still.
Then there’s tetrataenite – a magnetic material found in meteorites. Containing iron and nickel (FeNi) in a tetragonal crystal structure, it’s formed in nature after having cooled incredibly slowly at just a few degrees over millions of years. With a theoretical BHmax of 335 kJ/m3, it looks promising especially as iron and nickel are so cheap. In 2022 researchers in the UK and Austria fabricated it for the first time here on Earth by adding phosphorus (Adv.Sci. 10 2204315). What’s more, they made the tetrataenite in just a few seconds – between 11 and 15 orders of magnitude faster than in nature. It’s not clear what coercivity could be achieved and it’s early days for this material.
If you can cope with a less expensive rare earth, then SmFeN, which consists of samarium, iron and nitrogen, is a well-proven material system and offers excellent coercivity. Nichia – a Japanese company not to be taken lightly – is already pursuing this technology. Samarium is much cheaper and in less demand than neodymium so could be a good alternative.
Attractive future
Which of these materials will succeed is not clear – and there are many others I haven’t mentioned. In the short term – over the next five years or so – neodymium magnets will continue to dominate the market it seems, with cerium closing the gap from above and ferrites from below. Quite simply, nothing seems able to challenge the dominance of neodymium.
Indeed, we have plentiful reserves of rare-earth elements: estimates suggests there are eight million tonnes of neodymium on the planet widely spread around the world, with big economic deposits in Vietnam, Russia, India, Australia and Europe. The conern, though, is that China has a 80–90% market share (the exact figure depends on how you look at the supply chain) and there are big geopolitical questions over supply and control. What happens, for example, if tariffs were ever introduced or if products containing magnets from China were one day banned from sale.
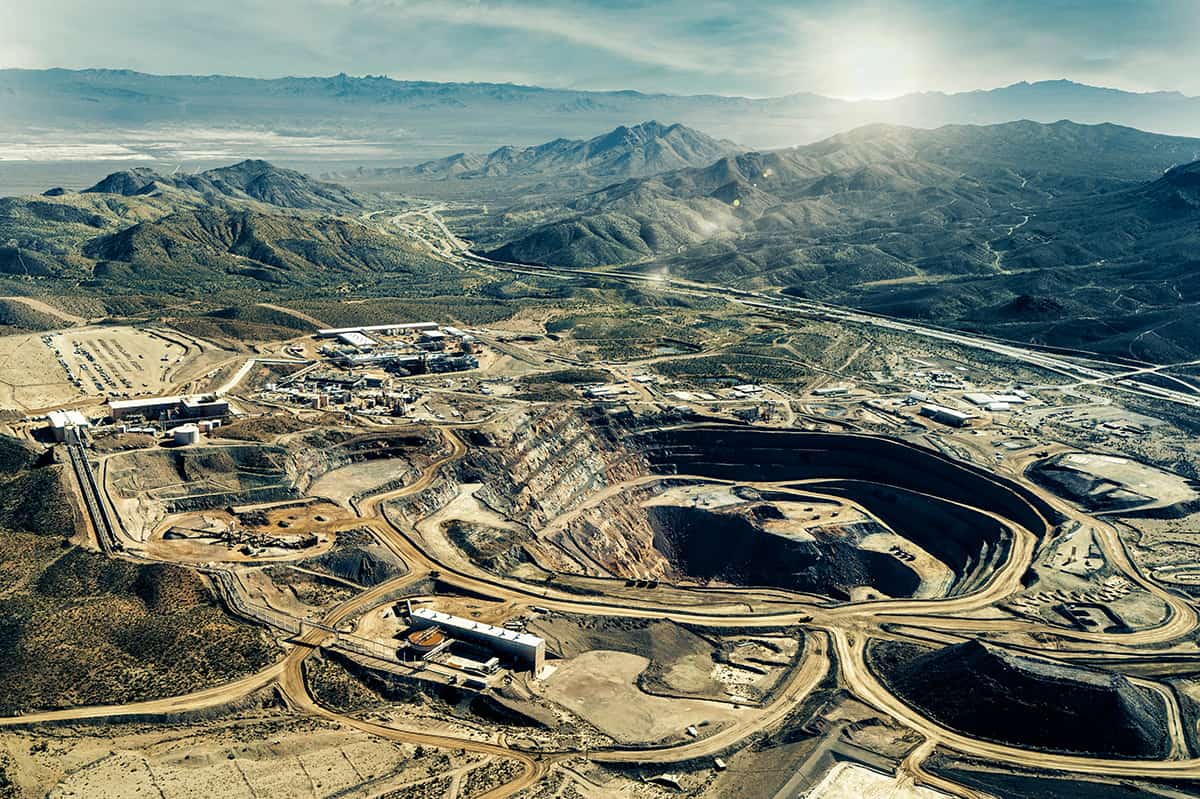
Such issues are naturally of great concern to companies making motors and generators, which is one reason why the US has been restoring its capabilities in rare-earth magnets. The Las-Vegas-based firm MP Materials, for example, is building a new processing facility for permanent-magnet materials at the Mountain Pass mine, which lies in California, near the border with Nevada. My view is that if the US manages to fully restore its own production of this material, concerns over supply will disappear entirely.
Longer term, though, the most promising alternatives are the “hard” ferrites, which are a much more environmentally friendly magnet and have the beauty of being available right here, right now. That, at least, seemed to be the consensus of the people I spoke to at the Birmingham meeting, with materials manufacturer Proterial (previously known as Hitachi Metals) having already built a 100 kW prototype ferrite magnet motor that’s suitable for electric vehicles.
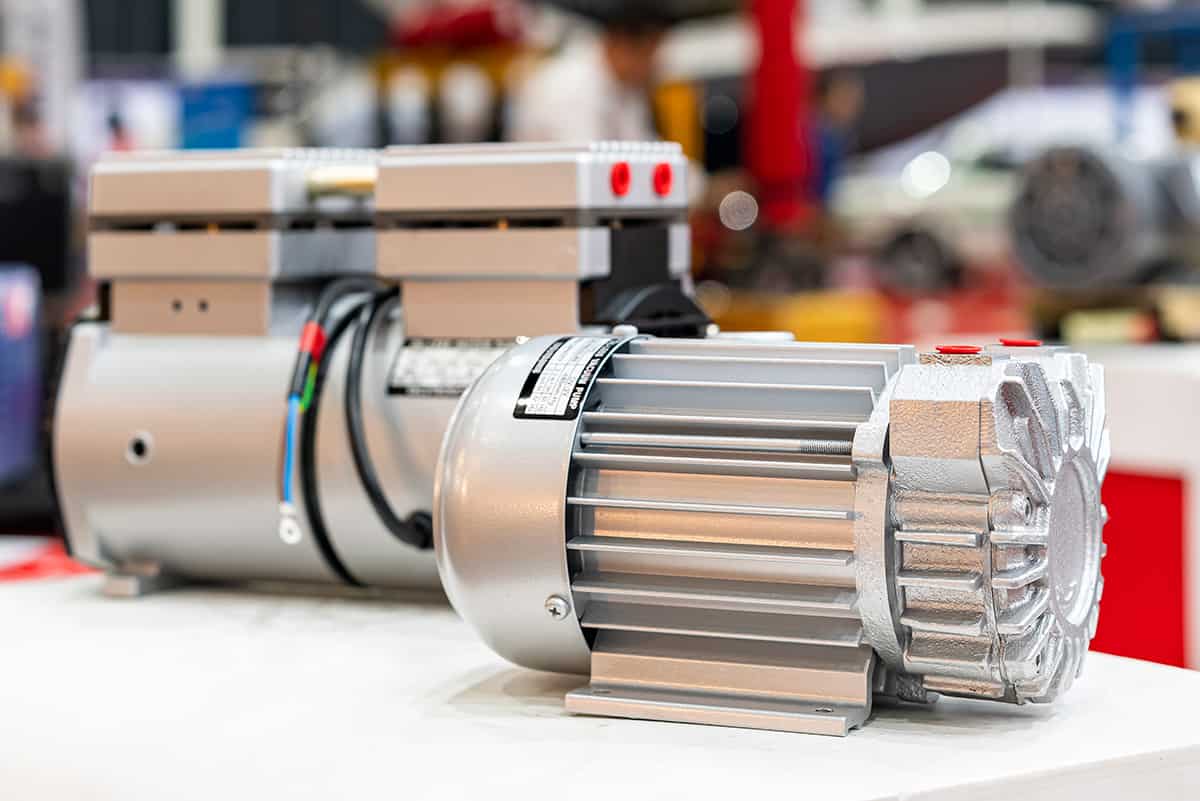
There is also the tantalizing prospect that we might not even need magnets for motors. Until recently, it was generally assumed that permanent magnetic motors were about 10% more efficient than conventional designs. But developments in power electronics and advances in the design of “induction” motors, which don’t have magnets at all, have closed this gap. In fact, some induction motors are on a par with those with permanent magnets, and although they are big and heavy, who knows what advances lie in store.
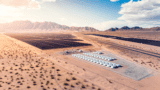
Here’s why Tesla’s Master Plan 3 makes a lot of sense for a sustainable future
In the meantime, those same advances in motor design and electronics mean that cheaper, less powerful magnet materials can be used. At the Birmingham meeting, everyone was talking about the latest master plan issued earlier this year by Tesla Motors, which contemplated eliminating rare earths entirely from its future permanent magnet motors. For the time being, though, with the help of advances in motor and generator design, the hard ferrites, I’d say, are the most promising alternative to rare earth magnets.
A world entirely free of rare-earths is, however, still a long way off.
- SEO Powered Content & PR Distribution. Get Amplified Today.
- PlatoData.Network Vertical Generative Ai. Empower Yourself. Access Here.
- PlatoAiStream. Web3 Intelligence. Knowledge Amplified. Access Here.
- PlatoESG. Carbon, CleanTech, Energy, Environment, Solar, Waste Management. Access Here.
- PlatoHealth. Biotech and Clinical Trials Intelligence. Access Here.
- Source: https://physicsworld.com/a/powering-the-green-economy-the-quest-for-magnets-without-rare-earths/
- :has
- :is
- :not
- :where
- $UP
- 000
- 11
- 15%
- 160
- 2021
- 2022
- 2030
- 27th
- 40
- 90
- a
- ability
- Able
- About
- above
- abundant
- abuse
- AC
- Academia
- According
- Accounting
- Achieve
- achieved
- active
- adding
- addition
- additives
- advances
- After
- All
- allows
- Alloy
- almost
- along
- already
- also
- alternative
- alternatives
- Although
- altogether
- amongst
- amount
- an
- analysis
- and
- applications
- ARE
- AREA
- around
- AS
- Assembly
- assumed
- At
- Australia
- available
- backed
- banned
- base
- based
- battery
- BE
- Beauty
- because
- been
- before
- being
- below
- Better
- between
- Big
- Birmingham
- border
- Building
- built
- but
- by
- california
- came
- CAN
- Can Get
- capabilities
- car
- carbon
- cars
- case
- chain
- challenge
- challenges
- cheap
- cheaper
- cheapest
- chemistry
- China
- chinese
- clear
- click
- closed
- closing
- CO
- comes
- commercial
- Communities
- community
- Companies
- company
- Concern
- Concerns
- conditions
- Congo
- Consensus
- consists
- contain
- contemplated
- continue
- continued
- control
- conventional
- Copper
- Cost
- costly
- Costs
- could
- country
- Crystal
- Current
- Currently
- day
- Days
- decades
- Demand
- democratic
- dependence
- depends
- deposits
- DESERT
- Design
- designing
- designs
- Despite
- developments
- Devices
- difficult
- DIG
- disappear
- discovered
- discussed
- Doesn’t
- Dominance
- dominate
- Dont
- Drop
- e
- Earlier
- Early
- earth
- Economic
- economy
- efficiency
- efficient
- effort
- either
- Electric
- electric car
- electric cars
- electric vehicle
- electric vehicles
- Electronics
- element
- elements
- eliminate
- eliminating
- elsewhere
- employing
- energy
- entirely
- environmental
- environmental concerns
- environmentally
- environmentally friendly
- especially
- essential
- essentially
- estimates
- Ether (ETH)
- ethical
- Europe
- Even
- EVER
- Every
- everyone
- everything
- example
- excellent
- exciting
- exist
- expansion
- expensive
- extra
- Facility
- fact
- factor
- factors
- faster
- featured
- few
- field
- Figure
- finding
- fine
- Firm
- firms
- First
- first time
- five
- For
- fore
- forgotten
- formed
- found
- four
- Free
- friendly
- from
- fully
- future
- gap
- General
- generally
- generator
- generators
- geopolitical
- get
- going
- good
- grand
- great
- Green
- Group
- Grow
- Growing
- happens
- Hard
- Have
- having
- Heart
- heavy
- Held
- help
- here
- hermes
- High
- high-performance
- higher
- highly
- hitachi
- Home
- Horizontal
- How
- However
- HTML
- HTTPS
- huge
- human
- human rights
- i
- if
- image
- importance
- important
- in
- Including
- incredibly
- india
- induction
- industry
- information
- interest
- International
- into
- introduced
- investing
- issue
- Issued
- issues
- IT
- ITS
- Japanese
- jpg
- just
- Key
- Kitco
- known
- knows
- large
- larger
- largest
- latest
- LEARN
- least
- Led
- less
- lie
- lies
- lightly
- like
- likely
- Limited
- Line
- Long
- Look
- LOOKS
- lose
- lost
- Lot
- lots
- love
- Low
- made
- Magnetic field
- Magnetism
- Magnets
- Main
- Mainstream
- make
- MAKES
- Making
- manages
- Manufacturer
- many
- Market
- masses
- master
- material
- materials
- Matter
- max-width
- maximum
- me
- mean
- means
- meantime
- Meanwhile
- measure
- meeting
- Men
- mentioned
- Merit
- Metals
- Meteorites
- might
- million
- millions
- minds
- mine
- mined
- minerals
- mines
- Mining
- money
- more
- more efficient
- most
- Motor
- Motors
- Mountain
- much
- my
- Nature
- Near
- Need
- needed
- needs
- NEVADA
- never
- New
- next
- Nickel
- no
- nothing
- now
- number
- occur
- of
- off
- Offers
- Officially
- on
- ONE
- only
- open
- opening
- optimizing
- or
- orders
- originally
- Other
- Others
- out
- Outperforms
- over
- overall
- own
- owned
- part
- particularly
- parts
- pass
- People
- people’s
- per
- perform
- performance
- periodic
- permanent
- Physics
- Physics World
- plan
- planet
- plato
- Plato Data Intelligence
- PlatoData
- Point
- Point of View
- poor
- potential
- power
- powerful
- Powering
- previously
- price
- Principal
- process
- processed
- processing
- produce
- Product
- Production
- Products
- promising
- properties
- prospect
- pursuing
- quest
- Questions
- quite
- RARE
- reach
- really
- reason
- recent
- recently
- Recover
- reduce
- reducing
- refined
- refining
- relatively
- replace
- report
- Reports
- Republic
- research
- reserves
- restore
- restoring
- right
- rights
- rising
- roughly
- running
- Russia
- s
- sale
- same
- say
- says
- SCI
- Scientist
- scientists
- Search
- seconds
- seeking
- seemed
- seems
- sense
- September
- set
- Sexual
- shocked
- Short
- showing
- similar
- simply
- site
- sits
- Size
- Slowly
- small
- So
- some
- something
- Source
- spent
- spikes
- Sport
- spread
- Still
- stopped
- store
- structure
- substantially
- succeed
- such
- Suggests
- suitable
- supply
- supply chain
- surging
- Surrounding
- sustainable
- system
- table
- Take
- taken
- talking
- tantalizing
- tariffs
- term
- terms
- Tesla
- than
- that
- The
- The Area
- the UK
- the world
- their
- Them
- then
- theoretical
- There.
- therefore
- These
- they
- this
- this year
- those
- though?
- thumbnail
- time
- to
- too
- trouble
- true
- trying
- Turning
- two
- types
- Uk
- uncertainties
- Uncertainty
- university
- until
- us
- use
- used
- value
- vehicle
- Vehicles
- very
- Vietnam
- View
- vital
- want
- was
- Way..
- we
- WELL
- were
- What
- when
- which
- WHO
- why
- widely
- Wikipedia
- will
- wind
- with
- without
- workers
- workshop
- world
- world’s
- worth
- would
- year
- years
- you
- Your
- zephyrnet