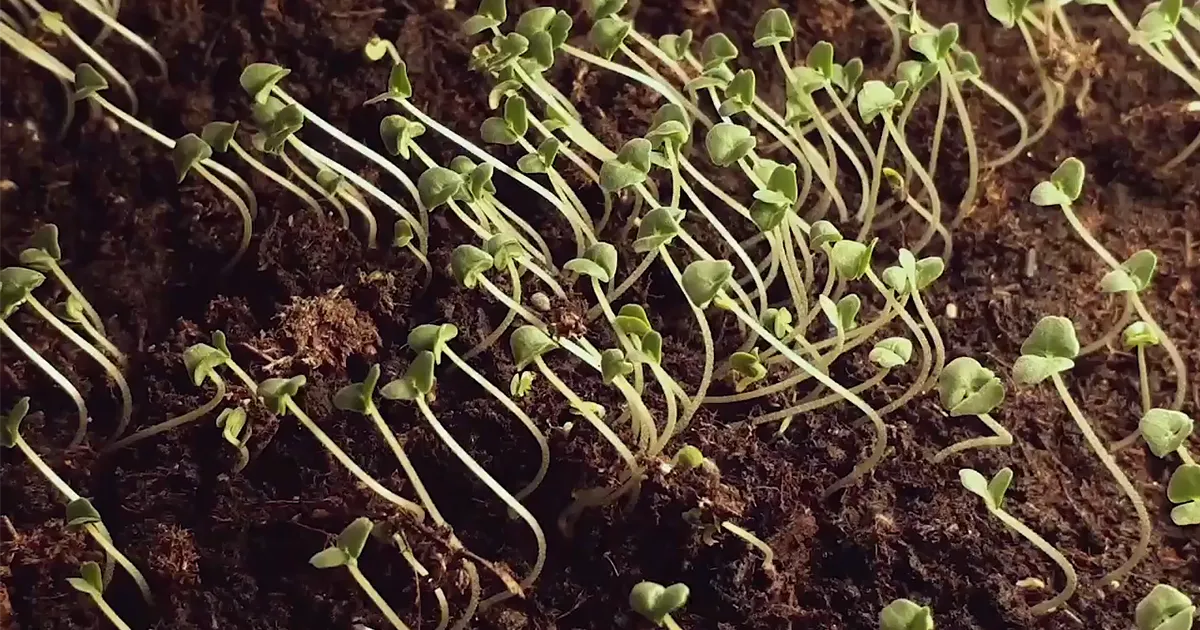
Introduction
On a shelf lined with terra cotta pots, herbs bend their stems toward the nearest window. In a field of golden wildflowers, leaves rotate with the path of the sun. In a dappled forest, vines twine up trees, reaching ever upward and away from the dark.
Since ancient times, plants’ ability to orient their eyeless bodies toward the nearest, brightest source of light — known today as phototropism — has fascinated scholars and generated countless scientific and philosophical debates. And over the past 150 years, botanists have successfully unraveled many of the key molecular pathways that underpin how plants sense light and act on that information.
Yet a critical mystery has endured. Animals use eyes — a complex organ of lenses and photoreceptors — to gain a detailed picture of the world around them, including the direction of light. Plants, biologists have established, possess a powerful suite of molecular tools for measuring illumination. But in the absence of obvious physical sensing organs like lenses, how do plants work out the precise direction from which light is coming?
Now, a team of European researchers has hit upon an answer. In a recent paper published in Science, they report that a roadside weed — Arabidopsis, a favorite of plant geneticists — uses the air spaces between its cells to scatter light, modifying the path of light passing through its tissues. In this way, the air channels create a light gradient that helps seedlings accurately determine where light is coming from.
By taking advantage of air channels to scatter light, plants sidestep the need for discrete organs like eyes in favor of a neater trick: the ability in effect to “see” with their whole bodies.
A Deep-Rooted Debate
Why and how plants orient themselves toward light has been the subject of fierce debate for well over 2,000 years. Early Greek philosophers argued that plants, like animals, were capable of sensation and movement, and even desire and intelligence. But later thinkers like Aristotle asserted that plants were innately passive, incapable of sensing their environment, much less moving with it. “Plants have neither sensation nor desire,” he wrote in On Plants. “These views we must repudiate as unsound.” For centuries, scholars tended to agree with him.
Introduction
It wasn’t until 1658 that the alchemist and natural philosopher Thomas Browne established phototropism as a fact by documenting that mustard seedlings growing in pots in a basement persistently oriented their growth toward an open window. But for more than two centuries thereafter, biologists continued to argue about how the plants did it, and whether they were responding to the sun’s light or its heat.
In 1880, Charles Darwin and his son Francis led experiments to describe a phototropic mechanism that was ultimately proved out. As described in The Power of Movement in Plants, the pair grew seedlings — plants that couldn’t yet perform photosynthesis, relying instead on stored energy from their seed — in a dark room. When blue light shone on them from a specific direction, the plants reached toward it. Then, as the Darwins moved the light around the room, they tracked the seedlings’ corresponding movements.
Based on their experiments, the Darwins suggested that seedlings were most light sensitive at the tip of the shoot, and that what they sensed there led to the production of some substance that influenced the direction of the plant’s growth. By the 1920s, botanists had settled on a comfortable consensus that elaborated on that model: that plants had light sensors at their tips and that they produced hormones (later identified as auxin) that encouraged more growth on their shaded sides, causing their stalks and leaves to bend toward light.
Like many great discoveries, this one opened up a new question: How exactly could plants sense light in the first place? They lacked any obvious sense organ. Researchers began to suspect that plants must have sophisticated sensory capabilities.
Molecular biologists took up the charge, showing that plants can measure and react to a far broader spectrum of light than we can with our animal eyes, even though they lack a specialized organ for perception. Five different families of photoreceptors, plus hormones and signal pathways, work together to dictate down to the cellular level the direction in which a plant builds new tissue — explaining how stems twist, turn and shoot upward as needed. These photoreceptors are spread throughout plant bodies but are largely concentrated in the inner tissue of the stem, said Christian Fankhauser, a plant biologist at the University of Lausanne in Switzerland and an author of the new study.
However, simple sensors aren’t enough on their own to give plants the ability to determine light’s direction. To best pinpoint the direction of strong illumination, a plant needs to be able to compare signals between different photoreceptors so that they can orient their growth toward the most intense light. And for that they need incoming light to fall onto their sensors in a gradient from brightest to dimmest.
Introduction
Animals have solved this problem through the development of eyes. A simple organism, such as a planarian worm, gets by with “eyespots” that merely sense the presence or absence of light. In more complex animal eyes like our own, anatomic features like the lens direct light toward the retina, which is packed with photosensors. The brain then compares the amount of light arriving through the curved lens with the amount registering on separate cells. This system, which combines physical manipulation of light with molecular sensors, allows for the detection of fine-grained gradients of brightness and shadow, and its resolution into the picture we call sight.
But since plants have no brain, they need a passive system for arriving at the same conclusions. That’s why plants’ ability to form physical gradients is important: They create inherent distinctions between cells without requiring the plant to make active comparisons.
Thus, botanists faced a conundrum. Was phototropism wholly a molecular process, as some suspected, or could plants alter light beams to create a gradient and better direct their response? If the latter was true, then plants must have physical structures that allow them to focus light.
That structure would finally be identified in a mutant version of a roadside weed that struggled to find the light.
The Blind Mutant
Thale cress — known to science as Arabidopsis thaliana — is not a particularly attractive plant. The 25-centimeter-tall weed is fond of disturbed land, field edges and the shoulders of roads. Native to Africa and Eurasia, it’s now found on every continent except Antarctica. Plant biologists have since adapted it to a scientific lifestyle: Its short life cycle, small genome (fully mapped in 2000) and tendency to produce useful mutations in the lab all make it an excellent model organism for understanding plant growth and genetics.
Fankhauser has worked with Arabidopsis since 1995 to study how light shapes plant growth. In 2016, his lab screened the genes of seedlings to find mutant plants with unusual responses to light. They grew the seeds in a dark room with blue lights to direct the seedlings sideways. From there, the experiment ran more or less as the Darwins’ did 150 years ago: As the researchers changed the direction of the light, the plants reoriented themselves to it.
However, one mutant plant struggled. While it had no problem sensing gravity, it seemed unable to track light. Instead it bent in all directions, as if blind and feeling around in the dark.
Something had apparently gone wrong with the mutant’s ability to sense light. When the team examined the plant, they found it had the typical photoreceptors, according to the plant biologist Martina Legris, a postdoc in Fankhauser’s lab and co-author on the new paper. But when the team looked at the stem under the microscope, they noticed something strange.
Introduction
The wild Arabidopsis, like most plants, has air channels between its cells. These structures are like ventilation shafts woven around the sealed cellular compartments, and they are known to play important roles both in photosynthesis and in oxygenating cells. But the air channels of the mutant plant were flooded with water. The team tracked the mutation to the gene abcg5, which produces a protein that may help waterproof the cell wall to ensure that the plant’s air shafts are watertight.
Intrigued, the researchers tried an experiment. They filled the intercellular air shafts of non-mutant plants with water to see whether this affected their growth. Like the mutants, these plants had a difficult time determining where the light was coming from. “We can see that these plants are genetically normal,” Legris said. “The only things they’re missing are these air channels.”
The researchers deduced that the plant orients itself to light through a mechanism based on the phenomenon of refraction — the tendency of light to change direction as it passes through different media. Because of refraction, Legris explained, light passing through a normal Arabidopsis will scatter under the surface of the stem: Every time it moves through a plant cell, which is mostly water, and then through an air channel, it changes direction. Since some of the light is redirected in the process, the air channels establish a steep light gradient across different cells, which the plant can use to assess the light’s direction and then grow toward it.
In contrast, when these air channels are filled with water, the scattering of light is reduced. Plant cells refract light in a similar way as a flooded channel, since they both contain water. Instead of scattering, the light passes almost straight through the cells and the flooded channels to deeper within the tissue, decreasing the light gradient and depriving the seedling of differences in light intensity.
Seeing the Light
The research suggests that these air channels play a critical role in helping young plants track light. Roger Hangarter, a plant biologist at Indiana University Bloomington, who was not involved in the new study, hailed it for finding a clever solution to a long-standing problem. Fankhauser, Legris and their colleagues “pretty well put the nail in the coffin on the importance of these air spaces,” he said.
The idea has come up before, Hangarter noted. In 1984, a team of researchers at the University of York suggested that air channels between plant cells might help establish the necessary light gradient. But since the team didn’t have the funding to carry out expensive experiments, their suggestion went untested.
“It was always baffling to us how these little, tiny — almost transparent — [embryonic plants] could detect a gradient,” Hangarter said. “We never really gave much credence to the air-space thing because we were distracted looking for molecules that were involved. You get on a certain research path, and you get blinders on.”
Introduction
The air-channel mechanism joins other ingenious devices that plants have evolved to control how light moves through them. For example, research by Hangarter helped establish that chloroplasts — the cellular organelles that perform photosynthesis — actively dance inside leaf cells to move light around. Chloroplasts can cluster greedily in the center of the cell to soak up weak light or flee to the margins to let stronger light pass deeper into plant tissues.
For now, the new findings about air channels extend only to seedlings. While these air channels also appear in adult leaves, where they’ve been shown to play a role in light scattering and distribution, nobody’s yet tested whether they play a role in phototropism, Legris said.
How long air channels have been playing this role is unclear. Primitive land-plant fossils from 400 million years ago show neither roots nor leaves — but the core tissues of the plants show quite large intercellular air spaces. Perhaps they arose initially for tissue aeration or gas exchange, Fankhauser said, and then were adapted to their role in phototropism. Or perhaps plants evolved air spaces in stems in part to help them sense light, and then co-opted them to perform other functions.
“Further understanding these structures — how they’re built, what is the mechanism behind them — is interesting for plant biologists beyond the question of how plants sense light direction,” Fankhauser said.
It could also help exorcise Aristotle’s ghost, which still lingers in people’s perceptions of plants, he said. “Many people have the feeling that plants are very passive organisms — they can’t anticipate anything; they just do what happens to them.”
But that idea is based in our expectations of what eyes should look like. Plants, it turns out, have evolved a way of seeing with their whole bodies, one woven into the gaps between their cells. They don’t need anything so clumsy as a pair of eyes to follow the light.
- SEO Powered Content & PR Distribution. Get Amplified Today.
- PlatoData.Network Vertical Generative Ai. Empower Yourself. Access Here.
- PlatoAiStream. Web3 Intelligence. Knowledge Amplified. Access Here.
- PlatoESG. Carbon, CleanTech, Energy, Environment, Solar, Waste Management. Access Here.
- PlatoHealth. Biotech and Clinical Trials Intelligence. Access Here.
- Source: https://www.quantamagazine.org/plants-find-light-using-gaps-between-their-cells-20240131/
- :has
- :is
- :not
- :where
- ][p
- $UP
- 000
- 150
- 1995
- 2011
- 2016
- 400
- a
- ability
- Able
- About
- According
- accurately
- across
- Act
- active
- adapted
- Adult
- ADvantage
- affected
- africa
- ago
- AIR
- All
- allow
- allows
- almost
- also
- always
- amount
- an
- Ancient
- and
- animal
- animals
- answer
- Antarctica
- anticipate
- any
- anything
- appear
- ARE
- argue
- argued
- around
- arriving
- AS
- assess
- At
- attractive
- author
- away
- based
- BE
- because
- been
- before
- began
- behind
- BEST
- Better
- between
- Beyond
- blind
- Blue
- bodies
- both
- Brain
- brightest
- broader
- builds
- built
- but
- by
- call
- CAN
- capabilities
- capable
- carry
- causing
- cell
- Cells
- cellular
- Center
- centuries
- certain
- change
- changed
- Changes
- Channel
- channels
- charge
- Charles
- Cluster
- Co-Author
- colleagues
- combines
- come
- comfortable
- coming
- compare
- comparisons
- complex
- Concentrated
- conclusions
- Consensus
- contain
- continent
- continued
- contrast
- control
- conundrum
- Core
- Corresponding
- could
- create
- critical
- cycle
- dance
- Dark
- debates
- decreasing
- deeper
- describe
- described
- desire
- detailed
- detect
- Detection
- Determine
- determining
- Development
- Devices
- dictate
- DID
- differences
- different
- difficult
- direct
- direction
- directions
- distribution
- do
- documenting
- Dont
- down
- Early
- effect
- elaborated
- encouraged
- energy
- enough
- ensure
- Environment
- establish
- established
- European
- Even
- EVER
- Every
- evolved
- exactly
- example
- excellent
- Except
- exchange
- expectations
- expensive
- experiment
- experiments
- explained
- explaining
- extend
- Eyes
- faced
- fact
- Fall
- families
- far
- favor
- Favorite
- Features
- feeling
- field
- fierce
- filled
- Finally
- Find
- finding
- findings
- First
- five
- flooded
- Focus
- follow
- For
- forest
- form
- Fossils
- found
- Francis
- from
- functions
- funding
- Gain
- gaps
- GAS
- gave
- generated
- Genetics
- genome
- get
- gets
- Ghost
- Give
- Golden
- gone
- gradients
- gravity
- great
- greek
- grew
- Grow
- Growing
- Growth
- had
- happens
- Have
- he
- help
- helped
- helping
- helps
- him
- his
- Hit
- How
- HTML
- http
- HTTPS
- idea
- identified
- if
- importance
- important
- in
- incapable
- Including
- Incoming
- Indiana
- influenced
- information
- inherent
- initially
- inner
- inside
- instead
- Intelligence
- intense
- interesting
- into
- involved
- IT
- ITS
- itself
- Joins
- just
- Key
- known
- lab
- Lack
- Land
- large
- largely
- later
- Led
- Lens
- lenses
- less
- let
- Level
- Life
- lifestyle
- light
- like
- lined
- little
- Long
- long-standing
- Look
- look like
- looked
- looking
- magazine
- make
- Manipulation
- many
- margins
- May..
- measure
- measuring
- mechanism
- Media
- merely
- Microscope
- might
- million
- missing
- model
- molecular
- more
- most
- mostly
- move
- moved
- movement
- movements
- moves
- moving
- much
- must
- Mutation
- Mystery
- native
- Natural
- necessary
- Need
- needed
- needs
- Neither
- never
- New
- no
- nor
- normal
- noted
- now
- obvious
- of
- on
- ONE
- only
- open
- opened
- or
- Other
- our
- out
- over
- own
- packed
- pair
- Paper
- part
- particularly
- pass
- passes
- Passing
- passive
- past
- path
- pathways
- People
- people’s
- perception
- perform
- perhaps
- persistently
- phenomenon
- Photosynthesis
- physical
- picture
- Place
- plant
- plants
- plato
- Plato Data Intelligence
- PlatoData
- Play
- playing
- plus
- possess
- power
- powerful
- precise
- presence
- primitive
- Problem
- process
- produce
- Produced
- produces
- Production
- Protein
- proved
- put
- Quantamagazine
- question
- reached
- reaching
- React
- really
- recent
- Reduced
- registering
- relying
- report
- research
- Research suggests
- researchers
- Resolution
- responding
- response
- responses
- roads
- Role
- roles
- Room
- roots
- Said
- same
- Scholars
- Science
- scientific
- see
- seed
- seeds
- seeing
- seemed
- sense
- sensitive
- sensors
- separate
- Settled
- Shadow
- shapes
- Shelf
- Shoot
- Short
- should
- shoulders
- show
- showing
- shown
- Sides
- sideways
- Sight
- Signal
- signals
- similar
- Simple
- since
- small
- So
- solution
- solved
- some
- something
- son
- sophisticated
- Source
- spaces
- specialized
- specific
- Spectrum
- spread
- Stem
- stems
- Still
- stored
- straight
- strange
- strong
- stronger
- structure
- structures
- Study
- subject
- substance
- Successfully
- such
- Suggests
- suite
- Sun
- Surface
- suspected
- switzerland
- system
- taking
- team
- Terra
- tested
- than
- that
- The
- the world
- their
- Them
- themselves
- then
- There.
- These
- they
- thing
- things
- thinkers
- this
- though?
- Through
- throughout
- time
- times
- tip
- tips
- tissue
- tissues
- to
- today
- together
- took
- tools
- toward
- track
- transparent
- Trees
- trick
- tried
- true
- TURN
- turns
- twist
- two
- typical
- Ultimately
- unable
- unclear
- under
- underpin
- understanding
- university
- until
- unusual
- upon
- upward
- us
- use
- useful
- uses
- using
- version
- very
- views
- Wall
- was
- Water
- watertight
- Way..
- we
- webp
- weed
- WELL
- went
- were
- What
- What is
- when
- whether
- which
- while
- WHO
- whole
- wholly
- why
- Wild
- will
- window
- with
- within
- without
- Work
- work out
- work together
- worked
- world
- worm
- would
- woven
- Wrong
- wrote
- X
- years
- yet
- york
- you
- young
- zephyrnet