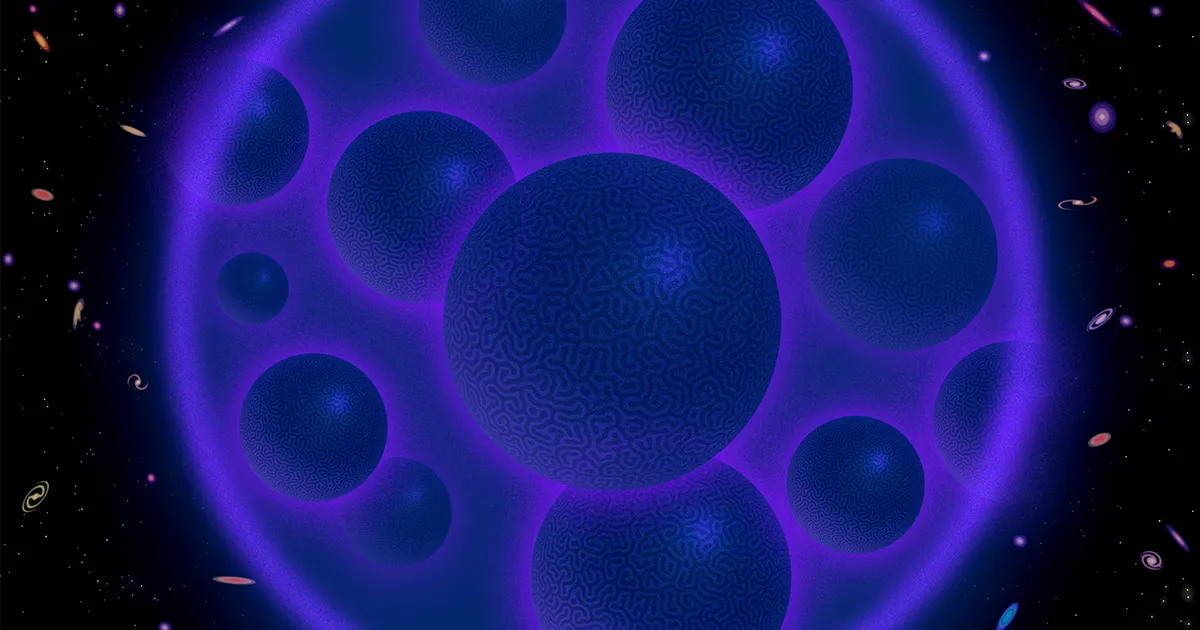
Introduction
When it comes to understanding the fabric of the universe, most of what scientists think exists is consigned to a dark, murky domain. Ordinary matter, the stuff we can see and touch, accounts for just 5% of the cosmos. The rest, cosmologists say, is dark energy and dark matter, mysterious substances that are labeled “dark” partly to reflect our ignorance about their true nature.
While no single idea is likely to explain everything we hope to know about the cosmos, an idea introduced two years ago could answer a few big questions. Called the dark dimension scenario, it offers a specific recipe for dark matter, and it suggests an intimate connection between dark matter and dark energy. The scenario might also tell us why gravity — which sculpts the universe on the largest scales — is so weak compared to the other forces.
The scenario proposes an as-yet-unseen dimension that lives within the already complex realm of string theory, which attempts to unify quantum mechanics and Einstein’s theory of gravity. In addition to the four familiar dimensions — three infinitely large spatial dimensions plus one of time — string theory suggests that there are six exceedingly tiny spatial dimensions.
In the dark dimension’s universe, one of those extra dimensions is significantly larger than the others. Instead of being 100 million trillion times smaller than the diameter of a proton, it measures about 1 micron across — minute by everyday standards, but enormous compared to the others. Massive particles that carry the gravitational force are generated within this dark dimension, and they make up the dark matter that scientists think comprises about 25% of our universe and forms the glue that keeps galaxies together. (Current estimates hold that the remaining 70% consists of dark energy, which is driving the universe’s expansion.)
The scenario “allows us to make connections between string theory, quantum gravity, particle physics and cosmology, [while] addressing some of the mysteries related to them,” said Ignatios Antoniadis, a physicist at Sorbonne University who is actively investigating the dark dimension proposal.
While there’s no evidence yet that the dark dimension exists, the scenario does make testable predictions for both cosmological observations and tabletop physics. That means we may not have to wait long to see whether the hypothesis will bear up under empirical scrutiny — or be relegated to the list of tantalizing ideas that never fulfilled their original promise.
“The dark dimension envisioned here,” said the physicist Rajesh Gopakumar, director of the International Center for Theoretical Sciences in Bengaluru, has “the virtue of being potentially ruled out fairly easily as upcoming experiments grow sharper.”
Divining the Dark Dimension
The dark dimension was inspired by a long-standing mystery concerning the cosmological constant — a term, designated by the Greek letter lambda, that Albert Einstein introduced into his equations of gravity in 1917. Believing in a static universe, as did many of his peers, Einstein added the term to keep the equations from describing an expanding universe. But in the 1920s, astronomers discovered that the universe is indeed swelling, and in 1998 they observed that it is growing at an accelerated clip, propelled by what is now commonly referred to as dark energy — which can also be denoted in equations by lambda.
Introduction
Since then, scientists have wrestled with one striking characteristic of lambda: Its estimated value of 10−122 in Planck units is “the smallest measured parameter in physics,” said Cumrun Vafa, a physicist at Harvard University. In 2022, while considering that almost unfathomable smallness with two members of his research team — Miguel Montero, now at Madrid’s Institute for Theoretical Physics, and Irene Valenzuela, currently at CERN — Vafa had an insight: Such a minuscule lambda is a truly extreme parameter, meaning it could be considered within the framework of Vafa’s previous work in string theory.
Earlier, he and others had formulated a conjecture that explains what happens when an important physical parameter takes on an extreme value. Called the distance conjecture, it refers to “distance” in an abstract sense: When a parameter moves toward the remote edge of possibility, thereby assuming an extreme value, there will be repercussions for the other parameters.
Thus, in the equations of string theory, key values — such as particle masses, lambda, or the coupling constants that dictate the strength of interactions — are not fixed. Altering one will inevitably affect the others.
For example, an extraordinarily small lambda, as has been observed, should be accompanied by much lighter, weakly interacting particles with masses directly linked to lambda’s value. “What could they be?” Vafa wondered.
As he and his colleagues pondered that question, they realized that the distance conjecture and string theory combined to provide one more key insight: For these lightweight particles to appear when lambda is almost zero, one of string theory’s extra dimensions must be significantly larger than the others — perhaps large enough for us to detect its presence and even measure it. They had arrived at the dark dimension.
The Dark Tower
To understand the genesis of the inferred light particles, we need to rewind cosmological history to the first microsecond after the Big Bang. At this time, the cosmos was dominated by radiation — photons and other particles moving close to the speed of light. These particles are already described by the Standard Model of particle physics, but in the dark dimension scenario, a family of particles that are not a part of the Standard Model can emerge when the familiar ones smash together.
“Every now and then, these radiation particles collided with each other, creating what we call ‘dark gravitons,’” said Georges Obied, a physicist at the University of Oxford who helped craft the theory of dark gravitons.
Normally, physicists define gravitons as massless particles that travel at the speed of light and convey the gravitational force, similar to the massless photons that convey the electromagnetic force. But in this scenario, as Obied explained, these early collisions created a different type of graviton — something with mass. More than that, they produced a range of different gravitons.
“There is one massless graviton, which is the usual graviton we know,” Obied said. “And then there are infinitely many copies of dark gravitons, all of which are massive.” The masses of the postulated dark gravitons are, roughly speaking, an integer times a constant, M, whose value is tied to the cosmological constant. And there’s a whole “tower” of them with a broad range of masses and energy levels.
To get a sense of how this all might work, imagine our four-dimensional world as the surface of a sphere. We cannot leave that surface, ever — for better or worse — and that’s also true for every particle in the Standard Model.
Gravitons, however, can go everywhere, for the same reason that gravity exists everywhere. And that’s where the dark dimension comes in.
To picture that dimension, Vafa said, think of every point on the imagined surface of our four-dimensional world and attach a small loop to it. That loop is (at least schematically) the extra dimension. If two Standard Model particles collide and create a graviton, the graviton “can leak into that extra-dimensional circle and travel around it like a wave,” Vafa said. (Quantum mechanics tells us that every particle, including gravitons and photons, can behave like both a particle and a wave — a 100-year-old concept known as wave-particle duality.)
As gravitons leak into the dark dimension, the waves they produce can have different frequencies, each corresponding to different energy levels. And those massive gravitons, traveling around the extra-dimensional loop, produce a significant gravitational influence at the point where the loop attaches to the sphere.
“Maybe this is the dark matter?” Vafa mused. The gravitons they had concocted were, after all, weakly interacting yet capable of mustering some gravitational heft. One merit of the idea, he noted, is that gravitons have been a part of physics for 90 years, having been first proposed as carriers of the gravitational force. (Gravitons, it should be noted, are hypothetical particles, and have not been directly detected.) To explain dark matter, “we don’t have to introduce a new particle,” he said.
Gravitons that can leak into the extra-dimensional domain are “natural candidates for dark matter,” said Georgi Dvali, director of the Max Planck Institute for Physics, who is not working directly on the dark dimension idea.
A large dimension such as the posited dark dimension would have room for long wavelengths, which imply low-frequency, low-energy, low-mass particles. But if a dark graviton leaked into one of string theory’s tiny dimensions, its wavelength would be exceedingly short and its mass and energy very high. Supermassive particles like this would be unstable and very short-lived. They “would be long gone,” Dvali said, “without having the possibility of serving as dark matter in the present universe.”
Gravity and its carrier, gravitons, permeate all the dimensions of string theory. But the dark dimension is so much bigger — by many orders of magnitude — than the other extra dimensions that the strength of gravity would get diluted, making it appear weak in our four-dimensional world, if it were seeping appreciably into the roomier dark dimension. “This explains the extraordinary difference [in strength] between gravity and the other forces,” said Dvali, noting that this same effect would be seen in other extra-dimensional scenarios.
Given that the dark dimension scenario can predict things like dark matter, it can be put to an empirical test. “If I give you some correlation you can never test, you can never prove me wrong,” said Valenzuela, a co-author of the original dark dimension paper. “It’s much more interesting to predict something that you can actually prove or disprove.”
Riddles of the Dark
Astronomers have known dark matter existed — at least in some form — since 1978, when the astronomer Vera Rubin established that galaxies were rotating so fast that stars on their outermost fringes would be cast off into the distance were it not for vast reservoirs of some unseen substance holding them back. Identifying that substance, however, has proved very difficult. Despite nearly 40 years of experimental efforts to detect dark matter, no such particle has been found.
If dark matter turns out to be dark gravitons, which are exceedingly weakly interacting, Vafa said, that won’t change. “They will never be found directly.”
But there may be opportunities to indirectly spot the signatures of those gravitons.
One strategy Vafa and his collaborators are pursuing draws on large-scale cosmological surveys that chart the distribution of galaxies and matter. In those distributions, there might be “small differences in clustering behavior,” Obied said, that would signal the presence of dark gravitons.
When heavier dark gravitons decay, they produce a pair of lighter dark gravitons with a combined mass that is slightly less than that of their parent particle. The missing mass is converted to kinetic energy (in keeping with Einstein’s formula, E = mc2), which gives the newly created gravitons a bit of a boost — a “kick velocity” that’s estimated to be about one-ten-thousandth of the speed of light.
These kick velocities, in turn, could affect how galaxies form. According to the standard cosmological model, galaxies start with a clump of matter whose gravitational pull attracts more matter. But gravitons with a sufficient kick velocity can escape this gravitational grip. If they do, the resulting galaxy will be slightly less massive than the standard cosmological model predicts. Astronomers can look for this difference.
Recent observations of cosmic structure from the Kilo-Degree Survey are so far consistent with the dark dimension: An analysis of data from that survey placed an upper bound on the kick velocity that was very close to the value predicted by Obied and his co-authors. A more stringent test will come from the Euclid space telescope, which launched last July.
Meanwhile, physicists are also planning to test the dark dimension idea in the laboratory. If gravity is leaking into a dark dimension that measures 1 micron across, one could, in principle, look for any deviations from the expected gravitational force between two objects separated by that same distance. It’s not an easy experiment to carry out, said Armin Shayeghi, a physicist at the Austrian Academy of Sciences who is conducting the test. But “there’s a simple reason for why we have to do this experiment,” he added: We won’t know how gravity behaves at such close distances until we look.
The closest measurement to date — carried out in 2020 at the University of Washington — involved a 52-micron separation between two test bodies. The Austrian group is hoping to eventually attain the 1-micron range predicted for the dark dimension.
While physicists find the dark dimension proposal intriguing, some are skeptical that it will work out. “Searching for extra dimensions through more precise experiments is a very interesting thing to do,” said Juan Maldacena, a physicist at the Institute for Advanced Study, “though I think that the probability of finding them is low.”
Joseph Conlon, a physicist at Oxford, shares that skepticism: “There are many ideas that would be important if true, but are probably not. This is one of them. The conjectures it is based on are somewhat ambitious, and I think the current evidence for them is rather weak.”
Of course, the weight of evidence can change, which is why we do experiments in the first place. The dark dimension proposal, if supported by upcoming tests, has the potential to bring us closer to understanding what dark matter is, how it is linked to both dark energy and gravity, and why gravity appears feeble compared to the other known forces. “Theorists are always trying to do this ‘tying together.’ The dark dimension is one of the most promising ideas I have heard in this direction,” Gopakumar said.
But in an ironic twist, the one thing the dark dimension hypothesis cannot explain is why the cosmological constant is so staggeringly small — a puzzling fact that essentially initiated this whole line of inquiry. “It’s true that this program does not explain that fact,” Vafa admitted. “But what we can say, drawing from this scenario, is that if lambda is small — and you spell out the consequences of that — a whole set of amazing things could fall into place.”
- SEO Powered Content & PR Distribution. Get Amplified Today.
- PlatoData.Network Vertical Generative Ai. Empower Yourself. Access Here.
- PlatoAiStream. Web3 Intelligence. Knowledge Amplified. Access Here.
- PlatoESG. Carbon, CleanTech, Energy, Environment, Solar, Waste Management. Access Here.
- PlatoHealth. Biotech and Clinical Trials Intelligence. Access Here.
- Source: https://www.quantamagazine.org/in-a-dark-dimension-physicists-search-for-missing-matter-20240201/
- :has
- :is
- :not
- :where
- ][p
- $UP
- 1
- 100
- 1998
- 2020
- 2022
- 40
- 90
- a
- About
- ABSTRACT
- AC
- Academy
- accelerated
- accompanied
- According
- Accounts
- across
- actively
- actually
- added
- addition
- addressing
- admitted
- advanced
- affect
- After
- ago
- All
- almost
- already
- also
- always
- amazing
- ambitious
- an
- analysis
- and
- answer
- any
- appear
- appears
- ARE
- around
- arrived
- AS
- At
- attach
- attain
- Attempts
- Attracts
- austrian
- back
- based
- BE
- Bear
- been
- behavior
- being
- believing
- Better
- between
- Big
- Big Bang
- bigger
- Bit
- bodies
- boost
- both
- bring
- broad
- but
- by
- call
- called
- CAN
- candidates
- cannot
- capable
- carried
- carriers
- carry
- Center
- CERN
- change
- characteristic
- Chart
- Circle
- Close
- closer
- clustering
- Co-Author
- collaborators
- colleagues
- Collide
- combined
- come
- comes
- commonly
- compared
- complex
- comprises
- concept
- concerning
- conducting
- conjecture
- connection
- Connections
- Consequences
- considered
- considering
- consistent
- consists
- constant
- converted
- copies
- Correlation
- Corresponding
- Cosmology
- Cosmos
- could
- course
- craft
- create
- created
- Creating
- Current
- Currently
- Dark
- Dark matter
- data
- define
- described
- describing
- designated
- Despite
- detect
- detected
- dictate
- DID
- difference
- differences
- different
- difficult
- diluted
- Dimension
- dimensions
- direction
- directly
- Director
- discovered
- distance
- distribution
- distributions
- do
- does
- domain
- dominated
- Dont
- drawing
- draws
- driving
- each
- Early
- easily
- easy
- Edge
- effect
- efforts
- einstein
- emerge
- energy
- enormous
- enough
- envisioned
- equations
- escape
- essentially
- established
- estimated
- estimates
- Even
- eventually
- EVER
- Every
- everyday
- everything
- everywhere
- evidence
- example
- existed
- exists
- expanding
- expansion
- expected
- experiment
- experimental
- experiments
- Explain
- explained
- Explains
- extra
- extraordinarily
- extraordinary
- extreme
- fabric
- fact
- fairly
- Fall
- familiar
- family
- far
- FAST
- few
- Find
- finding
- First
- fixed
- For
- Force
- Forces
- form
- forms
- formula
- found
- four
- Framework
- from
- Galaxies
- Galaxy
- generated
- Genesis
- get
- Give
- gives
- Go
- gone
- gravitational
- gravity
- greek
- Group
- Grow
- Growing
- had
- happens
- harvard
- harvard university
- Have
- having
- he
- heard
- helped
- here
- High
- his
- history
- hold
- holding
- hope
- hoping
- How
- However
- HTTPS
- i
- idea
- ideas
- identifying
- if
- Ignorance
- imagine
- imagined
- important
- in
- Including
- indeed
- indirectly
- inevitably
- inferred
- influence
- initiated
- inquiry
- insight
- inspired
- instead
- Institute
- interacting
- interactions
- interesting
- International
- intimate
- into
- intriguing
- introduce
- introduced
- involved
- ironic
- IT
- ITS
- July
- just
- Keep
- keeping
- Key
- kick
- Know
- known
- laboratory
- large
- large-scale
- larger
- largest
- Last
- launched
- leak
- leaking
- least
- Leave
- less
- letter
- levels
- light
- lighter
- lightweight
- like
- likely
- Line
- linked
- List
- Lives
- Long
- long-standing
- Look
- Low
- magazine
- make
- Making
- many
- Mass
- masses
- massive
- Matter
- max
- May..
- me
- meaning
- means
- measure
- measured
- measurement
- measures
- mechanics
- Members
- Merit
- micron
- might
- million
- minute
- missing
- model
- more
- most
- moves
- moving
- much
- must
- mysterious
- Mystery
- Nature
- nearly
- Need
- never
- New
- newly
- no
- noted
- noting
- now
- objects
- observations
- observed
- of
- off
- Offers
- on
- ONE
- ones
- opportunities
- or
- orders
- ordinary
- original
- Other
- Others
- our
- out
- Oxford
- pair
- parameter
- parameters
- part
- particle
- peers
- perhaps
- Photons
- physical
- Physical Parameter
- physicist
- Physics
- picture
- Place
- planning
- plato
- Plato Data Intelligence
- PlatoData
- plus
- Point
- possibility
- potential
- potentially
- precise
- predict
- predicted
- Predictions
- Predicts
- presence
- present
- previous
- principle
- probability
- probably
- produce
- Produced
- Program
- promise
- promising
- propelled
- proposal
- proposed
- proposes
- Prove
- proved
- provide
- pursuing
- put
- Quantum
- Quantum Mechanics
- question
- Questions
- Radiation
- range
- rather
- realized
- realm
- reason
- recipe
- referred
- refers
- reflect
- related
- remaining
- remote
- repercussions
- research
- REST
- resulting
- Rewind
- Room
- roughly
- ruled
- Said
- same
- say
- scales
- scenario
- SCIENCES
- scientists
- scrutiny
- Search
- see
- seen
- sense
- serving
- set
- Shares
- Short
- should
- Signal
- Signatures
- significant
- significantly
- similar
- Simple
- since
- single
- SIX
- skeptical
- Skepticism
- small
- smaller
- Smash
- So
- so Far
- some
- something
- somewhat
- Space
- space telescope
- Spatial
- speaking
- specific
- speed
- SPELL
- sphere
- Spot
- standard
- standards
- Stars
- start
- Strategy
- strength
- String
- stringent
- structure
- Study
- substance
- such
- sufficient
- Suggests
- Supported
- Surface
- Survey
- takes
- tantalizing
- team
- telescope
- tell
- tells
- term
- test
- tests
- than
- that
- The
- their
- Them
- then
- theoretical
- theory
- There.
- thereby
- These
- they
- thing
- things
- think
- this
- those
- three
- Through
- Tied
- time
- times
- to
- together
- touch
- toward
- travel
- Traveling
- Trillion
- true
- truly
- trying
- TURN
- turns
- twist
- two
- type
- under
- understand
- understanding
- units
- Universe
- university
- University of Oxford
- university of washington
- until
- upcoming
- us
- usual
- value
- Values
- Vast
- VeloCity
- very
- wait
- was
- washington
- Wave
- wavelengths
- waves
- we
- webp
- weight
- were
- What
- What is
- when
- whether
- which
- while
- WHO
- whole
- whose
- why
- will
- with
- within
- Work
- work out
- working
- world
- worse
- would
- Wrong
- years
- yet
- you
- zephyrnet
- zero